Spectroscopy for Materials Characterization
Здесь есть возможность читать онлайн «Spectroscopy for Materials Characterization» — ознакомительный отрывок электронной книги совершенно бесплатно, а после прочтения отрывка купить полную версию. В некоторых случаях можно слушать аудио, скачать через торрент в формате fb2 и присутствует краткое содержание. Жанр: unrecognised, на английском языке. Описание произведения, (предисловие) а так же отзывы посетителей доступны на портале библиотеки ЛибКат.
- Название:Spectroscopy for Materials Characterization
- Автор:
- Жанр:
- Год:неизвестен
- ISBN:нет данных
- Рейтинг книги:5 / 5. Голосов: 1
-
Избранное:Добавить в избранное
- Отзывы:
-
Ваша оценка:
- 100
- 1
- 2
- 3
- 4
- 5
Spectroscopy for Materials Characterization: краткое содержание, описание и аннотация
Предлагаем к чтению аннотацию, описание, краткое содержание или предисловие (зависит от того, что написал сам автор книги «Spectroscopy for Materials Characterization»). Если вы не нашли необходимую информацию о книге — напишите в комментариях, мы постараемся отыскать её.
Learn foundational and advanced spectroscopy techniques from leading researchers in physics, chemistry, surface science, and nanoscience Spectroscopy for Materials Characterization,
Simonpietro Agnello
Spectroscopy for Materials Characterization
Spectroscopy for Materials Characterization — читать онлайн ознакомительный отрывок
Ниже представлен текст книги, разбитый по страницам. Система сохранения места последней прочитанной страницы, позволяет с удобством читать онлайн бесплатно книгу «Spectroscopy for Materials Characterization», без необходимости каждый раз заново искать на чём Вы остановились. Поставьте закладку, и сможете в любой момент перейти на страницу, на которой закончили чтение.
Интервал:
Закладка:
The typical photocycle of CDs begins with the instantaneous photogeneration of an electron–hole couple where the electron is exposed to the environment on the surface, while the hole remains inside the core of the nanoparticle [70]. The surface electron first undergoes strong solvent‐induced relaxation on femtosecond and picosecond timescales and, at longer times (in the nanosecond range) the exciton recombines radiatively producing the fluorescence. In the presence of even small amounts (μM) of transition metal ions, CDs and ions form stable complexes wherein the fluorescence is strongly quenched. Under the static quenching regime (i.e. quenching of the emission without a variation of the nanosecond lifetime), TA experiments at different concentrations of copper ions were performed in order to clarify the ultimate mechanism responsible for fluorescence deactivation [29].
The TA signal of pure CDs ( Figure 3.9a) is characterized at all delays by three contributions: a negative contribution around the pump wavelength, mostly due to ground state bleaching (GSB) associated with the depopulation of the ground state via photoexcitation; a strong stimulated emission (SE) at 520–550 nm, spectrally matching the ordinary fluorescence signal except for its negative sign; and several positive excited state absorption (ESA) signals due to electronic transitions from the excited state toward higher excited states.
The spectral evolution of the signal entirely occurs in the first few picoseconds. The analysis of the observed dynamics shows that the kinetics are dominated by a progressive Stokes shift with negligible signal decay, as can be seen when comparing the peak position of the SE signal at two different times ( Figure 3.9a). This behavior can be attributed to excited state solvation, as well‐known for other systems [3, 4], and a detailed analysis of data in Figure 3.9a shows that the solvation timescales for CDs are 0.19 ps and 2.1 ps. Adding the external agent in solution (i.e. copper ions, Figure 3.9b) does not modify the shape of the signal. However, the entire signal now undergoes a progressive decay (compare the amplitude of the two signals in Figure 3.9b). Notably, a detailed analysis shows that the two timescales of this decay are found identical to those of solvation.
This result is consistent with a model in which fluorescence quenching is caused by an electron transfer from the CD to the ions: the consequent loss of correlation between electron and hole hinders radiative recombination causing the deactivation of the fluorescence, which we see in real time as a progressive decay of the SE signal in Figure 3.9b. Interestingly, the result that this electron transfer occurs bi‐exponentially on the same timescales of aqueous solvation, 0.19 and 2.1 ps, suggests that the photochemical electron transfer reaction is essentially driven by solvation. In this picture, while solvent molecules rearrange around the surface of the photoexcited CD–ion complex, on picosecond and sub‐picosecond timescales, they are responsible for driving the photoexcited system from the initial state to a final one in which the surface electron has been entirely transferred to the interacting metal ion, so destroying the e–h pair and fully quenching the emission.
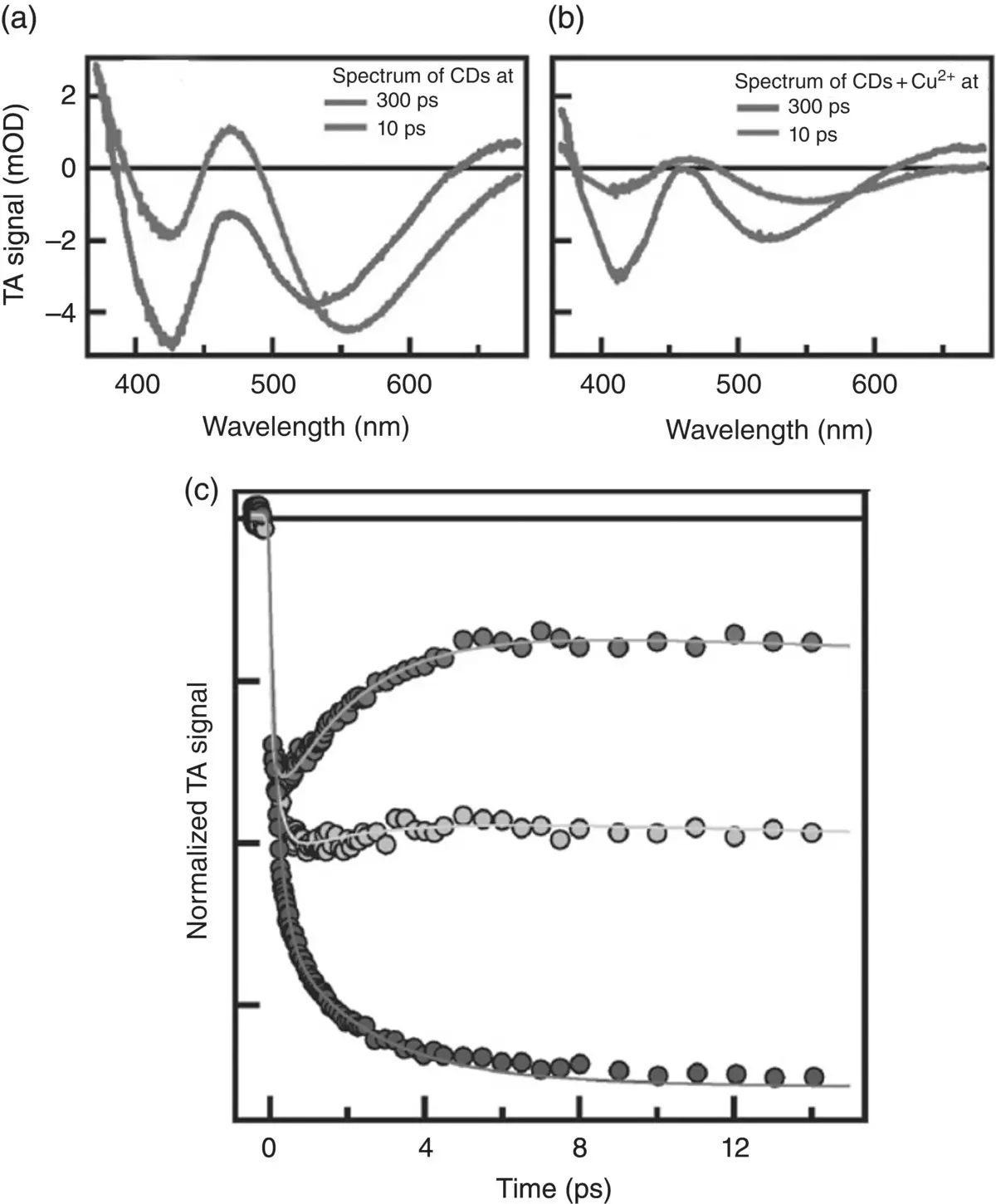
Figure 3.9TA spectra of bare CDs (a) and with 100 mM of Cu 2+(b) recorded at 300 fs and 10 ps after the photoexcitation. (c) Normalized kinetics traces at the SE signal with different amounts of copper ions (0, 20 mM, 100 mM).
Source: [29]. Adapted by permission of the Royal Society of Chemistry.
References
1 1 Rosspeintner, A., Lang, B., and Vauthey, E. (2013). Ultrafast photochemistry in liquids. Annu. Rev. Phys. Chem. 64: 247–271.
2 2 Cannizzo, A., Bräm, O., Zgrablic, G. et al. (2007). Femtosecond fluorescence upconversion setup with broadband detection in the ultraviolet. Opt. Lett. 32 (24): 3555–3557.
3 3 Jimenez, R., Fleming, G.R., Kumar, P.V., and Maroncelli, M. (1994). Femtosecond solvation dynamics of water. Nature 369: 471–473.
4 4 Pal, S.K., Peon, J., and Zewail, A.H. (2002). Biological water at the protein surface: dynamical solvation probed directly with femtosecond resolution. Proc. Natl. Acad. Sci. 99: 1763–1768.
5 5 Iwai, S., Murata, S., and Tachiya, M. (1998). Ultrafast fluorescence quenching by electron transfer and fluorescence from the second excited state of a charge transfer complex as studied by femtosecond up‐conversion spectroscopy. J. Chem. Phys. 109 (14): 5963–5970.
6 6 Marie, X. and Urbaszek, B. (2015). Ultrafast exciton dynamics. Nat. Mater. 14: 860–861.
7 7 Huber, R., Sp'orlein, S., Moser, J.E. et al. (2000). The role of surface states in the ultrafast photoinduced electron transfer from sensitizing dye molecules to semiconductor colloids. J. Phys. Chem. B 104: 8995–9003.
8 8 Klimov, V.I. (2007). Spectral and dynamical properties of multiexcitons in semiconductor nanocrystals. Annu. Rev. Phys. Chem. 58: 635–673.
9 9 Betz, M., Göger, G., Leitenstorfer, A. et al. (1999). Ultrafast electron‐phonon scattering in semiconductors studied by nondegenerate four‐wave mixing. Phys. Rev. B 60: R11265–R11268.
10 10 Saule, T., Heinrich, S., Schötz, J. et al. (2019). High‐flux ultrafast extreme‐ultraviolet photoemission spectroscopy at 18.4 mhz pulse repetition rate. Nat. Commun. 10: 458.
11 11 Mitrano, M., Lee, S., Husain, A.A. et al. (2019). Ultrafast time‐resolved x‐ray scattering reveals diffusive charge order dynamics in la2–xbaxcuo4. Sci. Adv. 5 (8).
12 12 Neppl, S., Mahl, J., Tremsin, A.S. et al. (2016). Towards efficient time‐resolved x‐ray absorption studies of electron dynamics at photocatalytic interfaces. Faraday Discuss. 194: 659–682.
13 13 Zhu, Y. and Cheng, J.‐X. (2020). Transient absorption microscopy: technological innovations and applications in materials science and life science. J. Chem. Phys. 152 (2): 020901.
14 14 Leone, S.R. and Neumark, D.M. (2016). Attosecond science in atomic, molecular, and condensed matter physics. Faraday Discuss. 194: 15–39.
15 15 Verma, S., Ghosh, A., Das, A., and Ghosh, H.N. (2010). Ultrafast exciton dynamics of j‐ and h‐aggregates of the porphyrin‐catechol in aqueous solution. J. Phys. Chem. B 114 (25): 8327–8334.
16 16 Strauss, V., Kahnt, A., Zolnhofer, E.M. et al. (2016). Assigning electronic states in carbon nanodots. Adv. Funct. Mater. 26 (44): 7975–7985.
17 17 Sciortino, A., Gazzetto, M., Buscarino, G. et al. (2018). Disentangling size effects and spectral inhomogeneity in carbon nanodots by ultrafast dynamical hole‐burning. Nanoscale 10: 15317–15323.
18 18 Fischer, A.J., Shan, W., Park, G.H. et al. (1997). Femtosecond four‐wave‐mixing studies of nearly homogeneously broadened excitons in Gan. Phys. Rev. B 56: 1077–1080.
19 19 Rullière, C. (2003). Femtosecond Laser Pulses Principles and Experiments. Springer.
20 20 Diels, J.‐C. and Rudolph, W. (2006). Ultrashort Laser Pulse Phenomena. Elsevier.
21 21 Abramczyk, H. (2005). Introduction to Laser Spectroscopy. Elsevier.
22 22 Weiner, A.M. (2009). Ultrafast Optics. Wiley.
23 23 Shah, J. (1988). Ultrafast luminescence spectroscopy using sum frequency generation. IEEE J. Quantum Electron 24 (2).
24 24 Di Carlo, S. and Messina, F. (2017). A collision timing monitor for superKEKB. Nucl. Inst. Methods Phys. Res. 869: 95–106.
25 25 Cerullo, G. and De Silvestri, S. (2002). Ultrafast optical parametric amplifiers. Rev. Sci. Instrum.: 74.
26 26 Alfano, R.R. (2006). The Supercontinuum Laser Source. Springer.
Читать дальшеИнтервал:
Закладка:
Похожие книги на «Spectroscopy for Materials Characterization»
Представляем Вашему вниманию похожие книги на «Spectroscopy for Materials Characterization» списком для выбора. Мы отобрали схожую по названию и смыслу литературу в надежде предоставить читателям больше вариантов отыскать новые, интересные, ещё непрочитанные произведения.
Обсуждение, отзывы о книге «Spectroscopy for Materials Characterization» и просто собственные мнения читателей. Оставьте ваши комментарии, напишите, что Вы думаете о произведении, его смысле или главных героях. Укажите что конкретно понравилось, а что нет, и почему Вы так считаете.