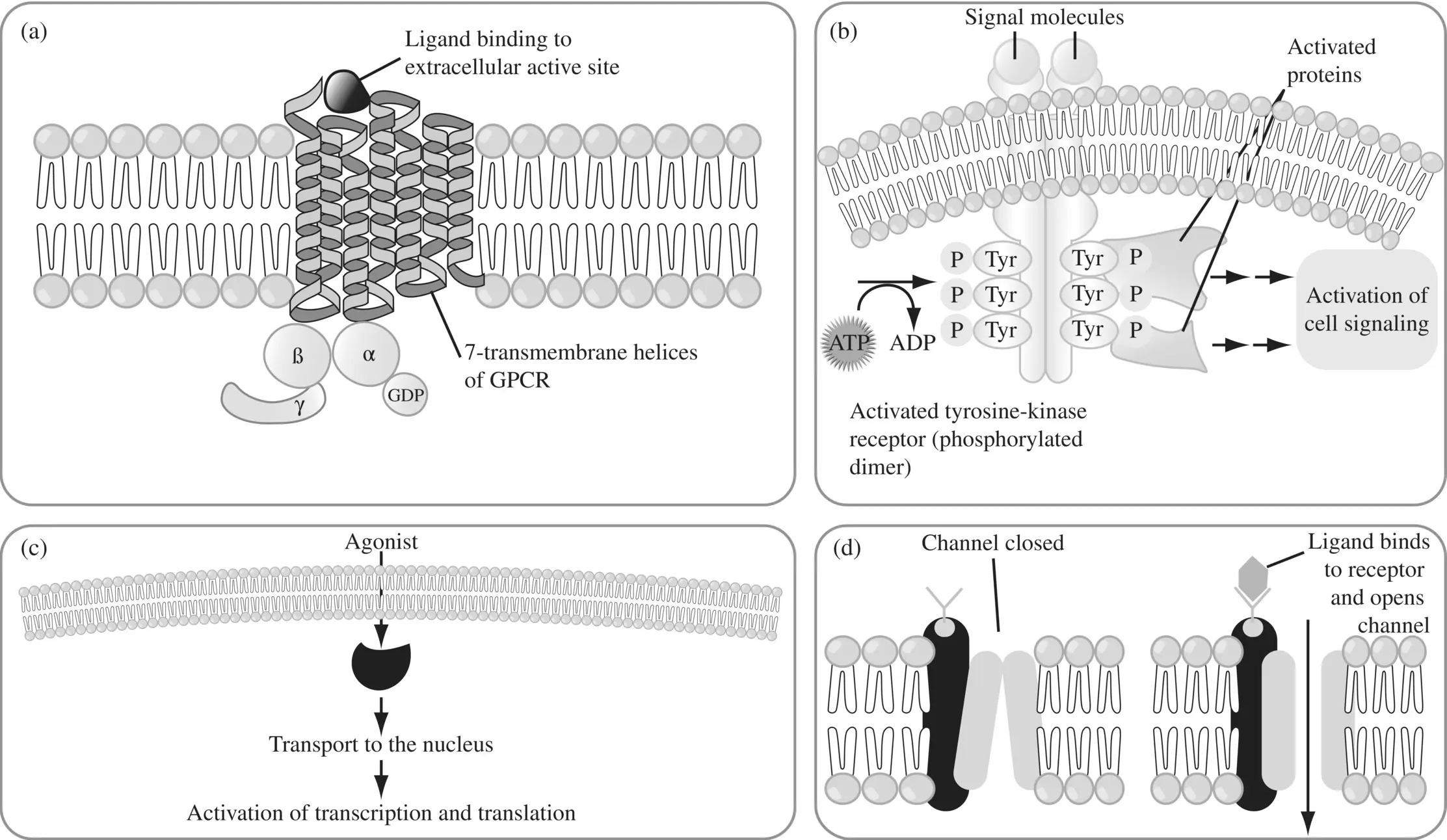
Figure 1.14. Target classes. (a) G‐protein‐coupled receptor (GPCR) (b) tyrosine kinase (c) nuclear receptor and (d) ion channel.
Most receptors can undergo agonist‐induced regulation. When an agonist occupies a receptor, it can result in a series of events that can lead to internalization of the receptor. The signals for internalization include phosphorylation of the receptor, which sets the stage for adapter molecules to bind to the receptor. The agonist is then removed, and the receptor is targeted for cycling back to the cell membrane or is trafficked into a degradation pathway. Cycling of the receptor is associated with resensitization of the receptor, while degradation results in a loss of total receptor density. When receptor density is decreased, it is usually associated with a shift to the right in the dose‐effect curve for agonists. An example of desensitization is the high affinity, desensitized, closed state of the nicotinic acetylcholine receptors (nAChRs), induced by chronic exposure to acetylcholine (ACh) or nicotinic drugs, leading to a gradual decrease in the rate of opening of K +, Na +, and sometimes Ca 2+cationic channels (milliseconds to minutes).
Sensitization has the opposite effect of tolerance, where an increase in drug effect is observed after repeated administration of certain drugs. When the body tries to return to homeostasis following a sudden discontinuation of a drug, the receptors which are deprived of their AGONISTs/blockers become hypersensitive to an agent that targets it, causing a further exacerbation of the symptoms/conditions that triggered the use of the drug in the first place. This rebound effect can be minimized by a gradual rather than a sudden discontinuation of the drug. Several anxiolytics and hypnotics have a rebound effect. For example, benzodiazepine withdrawal can cause severe anxiety and insomnia, worse than the original insomnia or anxiety disorder. Other examples causing rebound effects include sedatives like lunesta and ambien, the short acting hypnotic, triazolam (due to its high potency and ultra‐short half‐life), stimulants such as methylphenidate or dextroamphetamine antidepressants such as SSRIs, and alpha‐2 adrenergic agents such as clonidine and guanfacine. Rebound on drug withdrawal can be a factor in the chronic use of medications and drug dependence, with patients taking the medications only to ward off withdrawal or rebound withdrawal effects.
Time‐dependent changes in drug action arising from desensitization, sensitization, and rebound cause a loss of consistency in the concentration–effect relationship with increasing number of doses, leading to the serious consequences of reduced efficacy or increased toxicity with time which can be disastrous for drugs with narrow therapeutic window.
1.5.3 Biomarkers, Surrogate Endpoints, and Clinical Endpoints
The pharmacological effect of a drug or the response that it evokes in a species can be quantified using a biomarker (biological marker). A biomarker is an indicator of some biological or pathogenic processes and may be a protein, metabolite, DNA or RNA measured in blood, urine or soft tissues. The nature of biomarkers depends on whether they are meant for early screening assays such as binding or cell‐based assays, or whether they are used later in the value chain in in vivo/ex vivo preclinical or clinical development. They can provide great predictive value if they reflect the mechanism of drug action (target‐site drug exposure, drug–target interaction, target activation, signal transduction, homeostatic feedback mechanisms in normal and disease populations) and if the biomarker levels needed to reach the desired pharmacological effect is known.
A biomarker classification based on mechanism of drug action and drug–disease interaction has been proposed (Danhof et al., 2005). Type 0 biomarkers (also called pharmacogenomic or predictive biomarkers ) are measurable DNA/RNA characteristics that identify a PK or PD related genotype/phenotype of an individual, which determines drug response in that individual. For example, mutation of the epidermal growth factor receptor ( EGFR ) gene is reported to be associated with clinical responsiveness to the EGFR kinase inhibitor, gefitinib. Phosphorylated‐ EGFR for gefitinib assessed by immunohistochemistry assays is a good example of type 0 biomarker. Other examples include phosphorylated‐CRKL for Gleevec and mRNA gene expression‐based biomarkers for anti‐cancer drugs in cell‐based assays. Type 1 biomarker is a measure of drug exposure (plasma or more PD‐relevant target tissue concentrations of a drug). Type 2 ‐ type 4 constitute target engagement or PD biomarkers at the different levels of PD modulation. Type 2 biomarkers reflect receptor occupancy and can be useful in cases where target occupancy correlates well with therapeutic response (Nordström et al., 1993). Type 3 biomarkers quantify target activation, which is determined by the intrinsic efficacy of the drug and receptor density. Intrinsic efficacy of the drug determines the extent of occupancy needed for target activation, while receptor density determines the system maximum, which, if different between sites, will determine the selectivity of drug action. An example of type 3 biomarkers is the quantitative electroencephalogram (EEG) parameters reflecting the OP 3opioid receptor activation for synthetic opioids (Van Der Graaf et al., 1997). Type 4 biomarkers refer to physiological measures in the integral biological system. Disease biomarkers serving as functional endpoints of disease progression (such as tumor size in cancer) at a physiological level constitute the type 5 biomarkers. Biomarkers need not be directly related to the clinical outcome. For example, tumor size reduction need not necessarily correlate to how well the patient feels. Those that are predictive of clinical outcome are considered as surrogate biomarkers . However, even generally accepted surrogate endpoints are unlikely to capture all the therapeutic benefits and the potential adverse effects that a drug will have in a diverse patient population (Lesko and AJ Atkinson, 2001). Accordingly, combinations of biomarkers will probably be needed to provide a more complete characterization of the spectrum of pharmacological response. A clinical endpoint (type 6 biomarker) is a characteristic or variable that reflects how a patient feels, functions, or survives and therefore, the ultimate measure of efficacy that quantifies the direct benefit to a patient. However, the long periods of time needed to achieve it make it an impractical measure during the short‐term clinical trials. Table 1.6distinguishes Type 5 biomarkers from surrogate markers and clinical endpoints. Clinical endpoints include binary outcomes like cardiovascular events (presence or absence of stroke, myocardial infarction), death, or no death etc. Depending on the type of drug, some biomarkers are more relevant and readily available (Peck et al., 2003) than others. Biomarkers enable the demonstration of target engagement, modulation of pathophysiology or disease process and clinical efficacy needed for proof of mechanism, proof of principle, and proof of concept respectively, either in preclinical or clinical phases of drug development (see Figure 1.15). A quantitative relationship of target‐relevant drug concentration to PD biomarkers and to efficacy (surrogate marker/clinical endpoint) or long‐term safety, when supported by biology (pathway, disease) or by statistical evidence (if pathway is unknown) can guide dose‐finding and aid translation of nonclinical findings to humans (see Figure 1.15).
Читать дальше