Substituting for E Husing Equation 1.28, and assuming systemic clearance ( CL IV) is driven by hepatic metabolism only,
(1.37b) 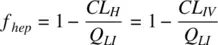
Knowing the F ( Equation 1.34) and f hep, the gut bioavailability ( f abs× f gut) of a drug may be estimated using Equation 1.36. Often, the IV PK may not be available, especially for poorly soluble drugs requiring separate formulations. These are costly to develop and may not be stable. Estimation of oral PK parameters in the absence of IV PK is described in Box 1.1. In the absence of IV PK, f hepmay be estimated by assuming complete drug absorption and neglecting gut metabolism, in which case the value of gut bioavailability becomes 1. In the absence of flip‐flop kinetics, the elimination rate and the half‐life may be obtained from the terminal slope of the oral PK profile. Obtaining clearance from f hep, the volume of distribution may be calculated. If gut metabolism cannot be neglected, then clearance can be calculated from the terminal half‐life, using a predicted volume of distribution.
1.2.6 Absorption from Solid Dosage Forms
An oral drug may be available in several dosage forms including the more common tablets and soft/hard gel capsules. A tablet comprises of the active pharmaceutical ingredient (API) and excipients compacted together and coated. Excipients include binders, flow‐aids, lubricants, preservatives, solubilizing, and flavoring agents. The release from these dosage forms and the dissolution of the drug may limit the rate of absorption.
The oral route is noninvasive and thus the most convenient route of drug administration. However, the bioavailability of many oral drugs is limited by drug solubility, dissolution, membrane permeability, or gut metabolism. Special formulations may therefore be needed to enhance drug exposure to the target tissue and control the exposure profile of a drug through the development of enabling and controlled‐release formulations respectively.
During drug development, the performance of different formulations, dosage forms, routes and different conditions of disease, fed/fasted states etc., may be compared even without IV PK, by determining the relative bioavailability, defined by Equation 1.38:
(1.38) 
Assuming that the IV clearance ( CL IV) is same in both conditions, Equation 1.38reduces to
(1.39) 
To ensure equivalent performance of different batches of drug formulations, regulatory agencies require bioequivalence studies to be performed. To establish bioequivalence, the 90% confidence interval about the geometric mean test/reference ratios for both AUC and C maxmust fall within the bioequivalence range, which is 80–125%.
1.2.7 Role of Transporters in ADME
Transporters ( Figure 1.7a) play an important role in enhancing or limiting the disposition of drugs (Ho and Kim, 2005) in plasma, target issue, as well as in blood–brain barrier (Shen and Zhang, 2010) as shown in Table 1.2. About 400 genes code for transporters in human and animals and these are classified into two large super‐families – the solute carrier or SLC superfamily containing 364 proteins in 48 subfamilies (Fredriksson et al., 2008) and the ATP‐binding cassette or ABC transporters of 49 proteins in 7 subfamilies. The SLC transporters mediate cellular influx of substrates either by facilitated diffusion or by secondary active transport, driven by co‐transport (symport or antiport) of endogenous organic ions (Zhang et al., 2002; Hediger et al., 2004; Hagenbuch and Gui, 2008; Duan and You, 2010). ABC transporters mediate the primary active transport of unidirectional efflux of drugs often against a steep diffusion gradient, deriving energy from ATP hydrolysis (Van De Water et al., 2005; Szakács et al., 2008; Schinkel and Jonker, 2012). The transporters that play an important role in human disposition are the active SLC transporters OATP1B1, 1B3 and 2B1, SLC transporters that mediate facilitated diffusion OCTs 1 and 2, SLC antiport transporters MATE1 and MATE2K, and the ABC efflux transporters P‐gp (MDR1), MRP2 (important in the transport of glucuronide and glutathione conjugates), BCRP and BSEP. ATP‐independent secondary efflux transporters are probably present an additional mechanism for toxin extrusion even if cells are energy‐deprived. The different types of transporters are shown in ( Figure 1.7b).
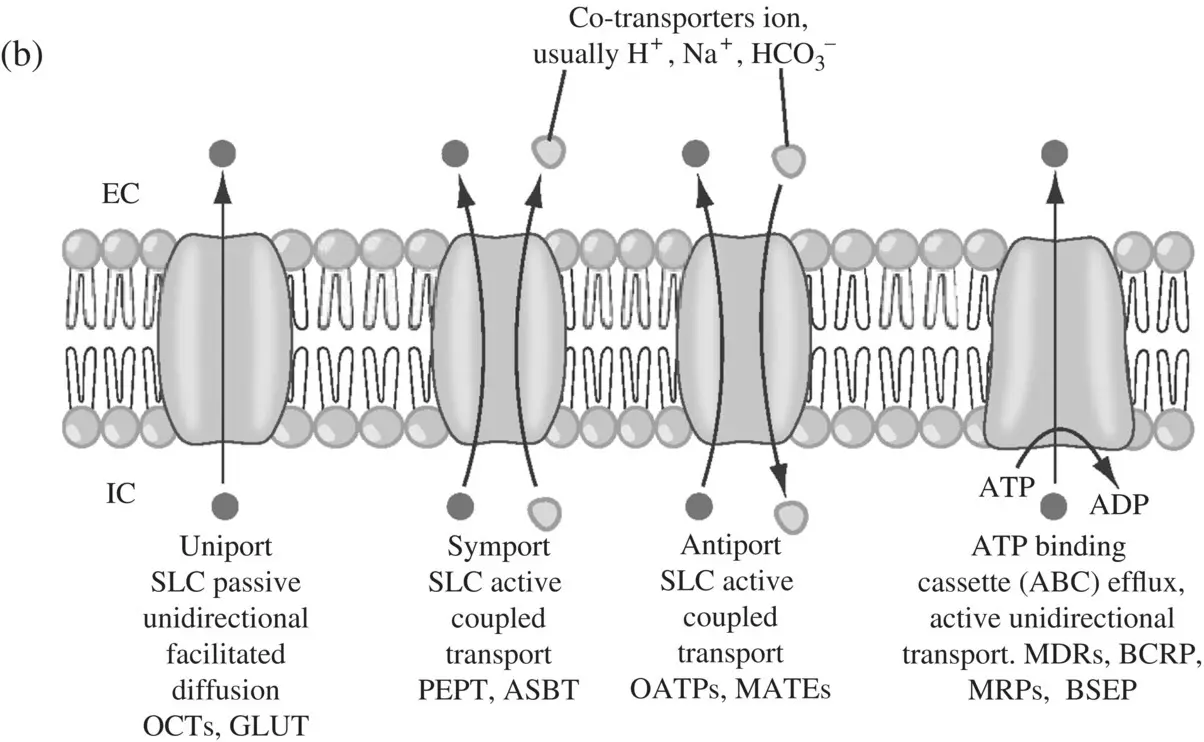
Figure 1.7. (a) Uptake and efflux drug transporters in intestine, liver, kidney, and brain. OAT, organic anion transporter; OATP, organic anion‐transporting polypeptide; NTCP, sodium taurocholate cotransporting peptide; OCT, organic cation transporter; OCTN, novel‐type OCT; PEPT, oligopeptide transporter; ASBT, apical sodium‐dependent bile acid transporter; MDR, multidrug‐resistant (or resistance); MRP, multidrug resistance‐associated protein; BCRP, breast cancer resistance protein; MATE, multidrug and toxin extrusion; MCT, monocarboxylate transporter; OST, organic solute transport; URAT, urate transporter. (b) Primary active ATP‐Binding Cassette (ABC) efflux transporters derive energy from the hydrolysis of ATP to ADP and are shown in dark shade. The secondary active transporter families or solute carrier (SLC) uptake transporters, utilize either ion gradients across membranes or cotransport with intracellular and/extracellular ions. These are either symports (unshaded) or antiports (lightly shaded). Multi‐drug efflux pump, MATE is a cation‐coupled family of transporters. OST α/β is a heterodimer that together transports bile acids, conjugated steroids, and structurally related molecules. Clinically relevant transporters are shown in bold (Source: Ho and Kim 2005).
TABLE 1.2. Role of transporters in ADME.
ADME |
Transporter |
Example |
Role |
Absorption |
Carriers |
PEPT |
Enhances absorption by transporting hydrophilic compounds with specific groups like peptide linkages |
Absorption |
Efflux |
P‐gp BCRP MRP2 |
Limits absorption of large lipophilic molecules. P‐gp has broad specificity |
Absorption |
Uptake |
OATP |
Uptake of organic ions |
Distribution |
Uptake, efflux |
Various |
Increases or decreases tissue distribution |
Metabolism |
Uptake |
OATP1B1 OATP1B3 OATP2B1 |
Increases or decreases exposure to drug‐metabolizing enzymes thereby increasing or decreasing metabolism |
Biliary elimination |
Efflux |
P‐gp BCRP |
Increases biliary elimination of lipophilic, amphiphilic compounds |
Renal elimination |
Uptake |
OAT 1,2,3 OCT2 OATP4C1 |
Increases uptake and elimination of hydrophilic compounds |
Renal elimination |
Efflux |
P‐gp, MATE1, MATE2‐K MRP2, 4 |
Removal (active secretion) of toxins |
The role of the efflux transporter P‐gp in restricting absorption, transporting amphiphilic compounds into bile and keeping out lipophilic compounds from the brain has long been recognized. It is difficult to predict the disposition of compounds that are transported, as the substrate specificity to most transporters are dependent on the chemical structure of the drug. However, there are some general principles for identifying transporter substrates (Wright and Dantzler, 2004; El‐Sheikh et al., 2008; Nies et al., 2008; Ahn and Nigam, 2009; Kusuhara and Sugiyama, 2009). For example, P‐gp substrates are mostly large and lipophilic. Substrates of the uptake transporter OATP1B1 are mostly carboxylic acids and so on. Efflux transporters like MRP2 and BCRP have similar substrate specificity to uptake transporters, reducing the possibility for toxin accumulation.
Читать дальше