Source: Adapted with permission from Ref. [42] (Copyright 2010 Royal Society of Chemistry).
Another type of a series of SSB probe is to detect hydrophobic proteins such as BSA and human serum albumin (HSA) in aqueous solution. Figure 3.17a shows a ratiometric fluorescent probe 29for the detection of hydrophobic proteins (casein) or proteins with hydrophobic pockets (BSA, HSA) through hydrophobic interaction [44]. Probe 29emits a blue fluorescence at 436 nm due to the deprotonation of the hydroxyl group when it dissolves in water at pH 7.4. When binding to the hydrophobic pocket of a protein such as BSA, the OH group recovers generating a new red‐shifted emission enhancement at 518 nm, resulting in an obvious fluorescent color distinction that can be easily distinguished by naked eye ( Figure 3.17b, c). The fluorescence intensity ratio, I 518/ I 436, was linearly related to the concentrations of a series of hydrophobic proteins. The detection limits for BSA, HSA, and casein based on IUPAC ( C DL= 3 Sb/m) were 16.2, 10.5, and 5.7 mg/ml, respectively.
Enzymes are biomacromolecules that accelerate or catalyze biological or chemical reactions, and most enzymes belong to an important class of proteins in essence. There is no doubt that enzymes play a critical part in nearly all metabolic processes so that the evaluation of enzyme activity is of great significance. A mostly general and direct idea for the enzyme activity detection probe design is to modify the functional group with a substrate of the enzyme to block the probe fluorescence. As shown in Figure 3.18a and d, substituting ortho ‐hydroxyl groups of salicylaldehyde to destroy the ESIPT process and quench fluorescence is a very simple and effective way to design fluorescence light‐up enzyme probes. Probes 30and 31are two examples following this design principle for β‐galactosidase and esterase activity evaluation, respectively [45, 47]. The probes perform good linear relationship at the range of 0–0.1 U/ml for β‐galactosidase and 0.01–0.15 U/ml for esterase, with the detection limits for β‐galactosidase and esterase as 0.014 and 0.005 U/ml, respectively. Such probes also perform well in imaging enzymes in live cells with low cytotoxicity.
Figure 3.17 (a) Synthesis and schematic presentation of the ratiometric fluorescence change of 29upon binding to the hydrophobic pocket of BSA. The emission wavelength of 29changes from 436 to 518 nm. (b) Photographs of 29before and after the addition of various kinds of proteins under a UV lamp (365 nm). (c) Fluorescence spectra of 29(10 mM) upon the addition of various concentrations of BSA in 10 mM PBS buffer at pH = 7.4. Excitation wavelength was set at 363 nm. (d) Ratiometric calibration curve of I 518/ I 436as a function of BSA concentrations.
Source: Reprinted from Ref. [44] (Copyright 2013 Royal Society of Chemistry).
There is also an indirect approach to detect enzymes like β‐lactamase, an important bacterial enzyme for some kinds of bacteria resistant to β‐lactam antibiotics (penicillins, cephalosporins, etc.), by cleaving the amide group with high catalytic efficiency [46]. The detection method contains three steps for fluorescence lighting‐up. As Figure 3.19a illustrates, β‐lactamase reacts with the lactam of its substrate (cefazolin sodium) to produce a secondary amine, initiating a spontaneous elimination reaction and affording a thiol compound. The thiol could further react with the sulfonate group of probe 32, releasing the SSB derivative with both AIE and ESIPT characteristics. The fluorescent signal enhancement relates linearly in the range of 0–10 mU/ml, and the detection limit was 0.5 mU/ml. This indirect method was also successfully applied to testing paper fabrication and achieved good analytical performance.
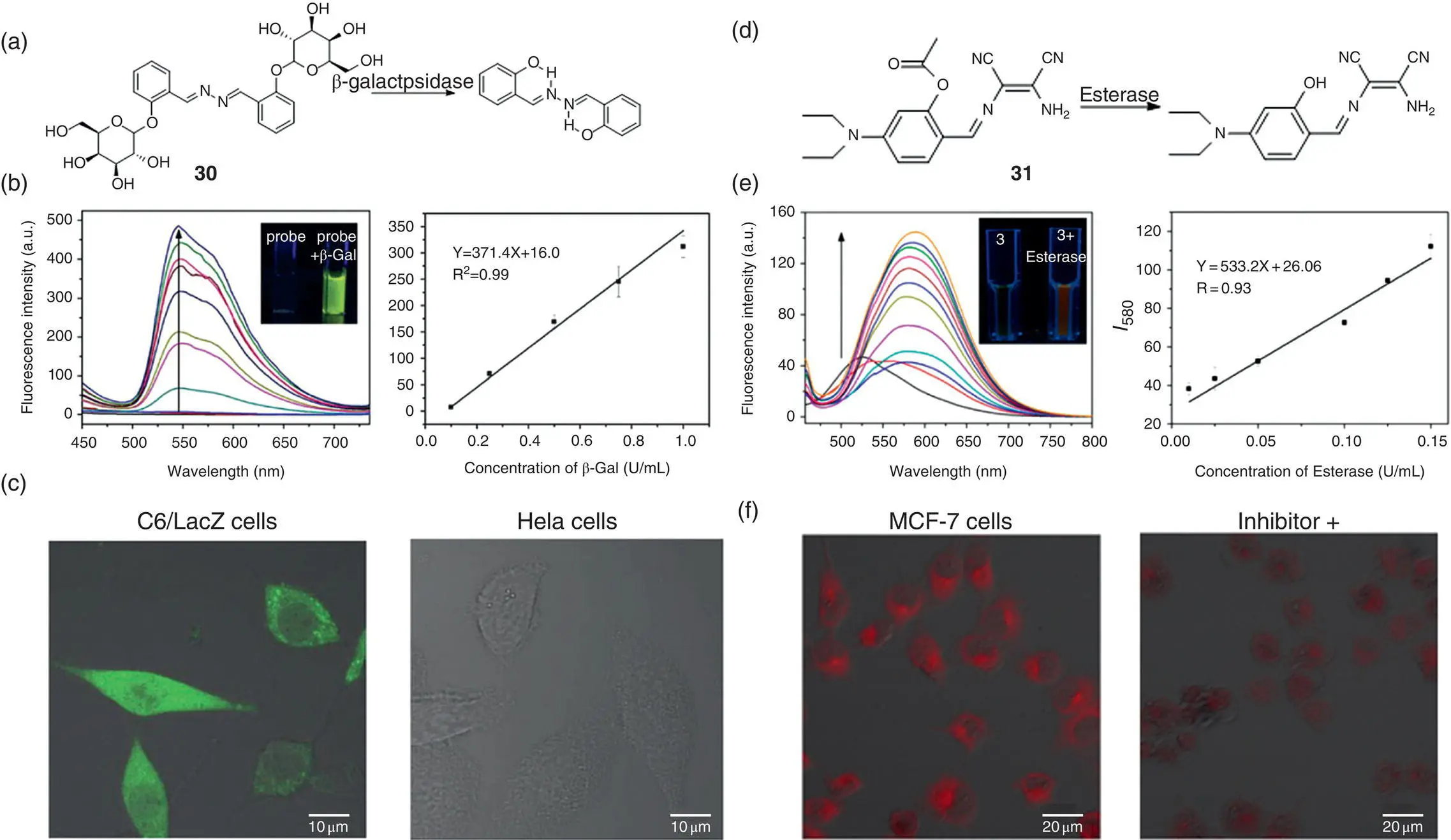
Figure 3.18 (a) Fluorescent light‐up probe 30for β‐galactosidase detection. (b) Fluorescence spectra of 30(100 μM) in the presence of various concentrations of β‐galactosidase in the PBS buffer solution and calibration curve of the fluorescence intensities ( I 545) versus β‐galactosidase concentrations. Insets from left to right: photographs of 30(100 μM) without or with β‐galactosidase under UV light (365 nm). (c) Imaging β‐galactosidase activity in cells. Images of probe 30(50 μM) in C6/LacZ cells and HeLa cells for two hours at 37 °C.
Source: Panels (a–c) are adapted permission from Ref. [45] (Copyright 2015 Royal Society of Chemistry).
(d) Fluorescent light‐up probe 31for sensing of esterase. (e) Fluorescence spectra of 31(100 μM) in the presence of various concentrations of esterase (0–1.0 U/ml) in a 10 mM PBS buffer solution and calibration curve of the fluorescence intensities ( I 580) versus esterase concentrations at pH 7.4, 37 °C. Insets from left to right: photographs of 31(100 μM) without or with esterase under UV light (365 nm). (f) Confocal fluorescent image of MCF‐7 cells with incubation of 31(50 μM) for 10 minutes or preincubated with a 10 mM inhibitor for 20 minutes and then treated with 31(50 μM) for 10 minutes.
Source: Panels (d–f) are reprinted from Ref. [47] (Copyright 2017 American Chemical Society).
Figure 3.19 (a) An indirect approach for fluorescence light‐up detection of β‐lactamase using probe 32. (b) Photographs of test papers under a UV lamp (365 nm) for the detection of β‐lactamase at various concentrations (0–7.0 mU/ml) in the PBS solution containing cefazolin sodium (4.8 mM). (c) The corresponding fluorescence intensity of spots read by Image J software versus the concentrations of β‐lactamase. (d) Calibration curve for β‐lactamase detection.
Source: Reprinted from Ref. [46] (Copyright 2018 Royal Society of Chemistry).
A polysaccharide SSB sensor for facile, sensitive, and selective heparin detection has also been fabricated [48]. Heparin is a mucopolysaccharide composed of D‐β‐glucuronic acid and N ‐acetylglucosamine to form a repeating disaccharide unit. Its skeleton has many anionic groups (such as carboxyl and sulfonic acid groups, etc.), making heparin highly negatively charged. As a medicinal anti‐hemagglutinating agent as well as a special antidote, heparin is hence of great significance for analytical detection. As shown in Figure 3.20a, salicylazine 33is modified with two positively charged tertiary amine groups, which can be combined with negatively charged heparin through a charge–charge interaction. The emission of probe 33in the Tris‐HCl buffer solution at pH 7.0 was extremely weak may be and its emission at 530 nm increased rapidly upon the addition of heparin ( Figure 3.20b), which was due to the aggregation through electrostatic interactions. When the concentration of heparin reached 22 μg/ml, a fluorescence enhancement of about 40‐folds had been detected. The linear range is 0.2–14 μg/ml, the detection limit is 57.6 ng/ml, and the response time is as short as 2 minutes. Figure 3.20c also represents good selectivity of probe 33for heparin from other polysaccharides such as chondroitin sulfate (ChS), hyaluronic acid (HA), and dextran (DeX).
Читать дальше