Mohamed N. Rahaman - Materials for Biomedical Engineering
Здесь есть возможность читать онлайн «Mohamed N. Rahaman - Materials for Biomedical Engineering» — ознакомительный отрывок электронной книги совершенно бесплатно, а после прочтения отрывка купить полную версию. В некоторых случаях можно слушать аудио, скачать через торрент в формате fb2 и присутствует краткое содержание. Жанр: unrecognised, на английском языке. Описание произведения, (предисловие) а так же отзывы посетителей доступны на портале библиотеки ЛибКат.
- Название:Materials for Biomedical Engineering
- Автор:
- Жанр:
- Год:неизвестен
- ISBN:нет данных
- Рейтинг книги:4 / 5. Голосов: 1
-
Избранное:Добавить в избранное
- Отзывы:
-
Ваша оценка:
- 80
- 1
- 2
- 3
- 4
- 5
Materials for Biomedical Engineering: краткое содержание, описание и аннотация
Предлагаем к чтению аннотацию, описание, краткое содержание или предисловие (зависит от того, что написал сам автор книги «Materials for Biomedical Engineering»). Если вы не нашли необходимую информацию о книге — напишите в комментариях, мы постараемся отыскать её.
A comprehensive yet accessible introductory textbook designed for one-semester courses in biomaterials Materials for Biomedical Engineering: Fundamentals and Applications
Materials for Biomedical Engineering: Fundamentals and Applications
Materials for Biomedical Engineering — читать онлайн ознакомительный отрывок
Ниже представлен текст книги, разбитый по страницам. Система сохранения места последней прочитанной страницы, позволяет с удобством читать онлайн бесплатно книгу «Materials for Biomedical Engineering», без необходимости каждый раз заново искать на чём Вы остановились. Поставьте закладку, и сможете в любой момент перейти на страницу, на которой закончили чтение.
Интервал:
Закладка:
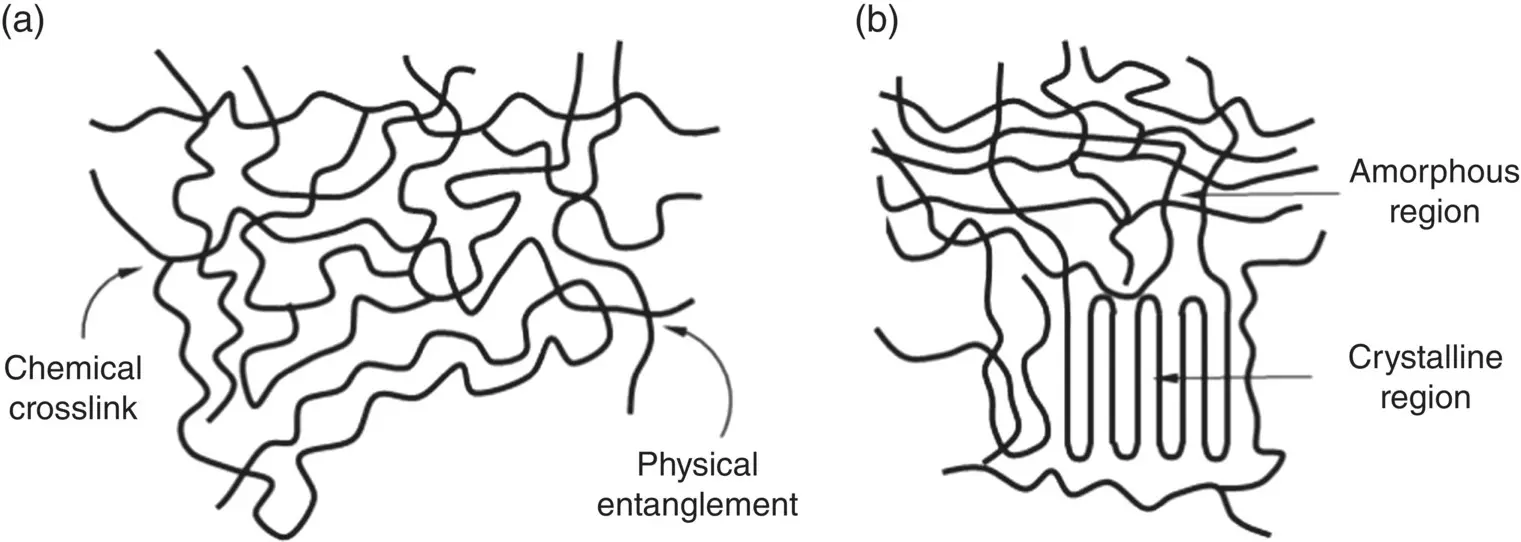
Figure 3.15 Schematic representation of (a) random arrangement of macromolecules in a polymer to give an amorphous structure and (b) ordered packing of macromolecule in crystalline regions of a semicrystalline polymer. For clarity, only the chain backbone of the macromolecules are shown.
Whether a polymer is amorphous or semicrystalline has significant consequences for its properties and applications. The crystalline regions typically have better mechanical properties than the amorphous regions, such as higher strength, higher elastic modulus and better wear resistance. Improvement in the percentage of crystalline regions in PE, for example, has resulted in reduced wear of ultrahigh molecular weight polyethylene (UHMWPE) bearings in hip and knee implants. In degradable polymers such as PLA and PGA, for example, the crystalline regions have a lower degradation rate than the amorphous regions. Consequently, amorphous copolymers of PLA and PGA, referred to as polylactic‐co‐glycolic acid (PLGA), are often preferred in drug delivery devices because they provide a more predictable degradation rate than semicrystalline PLA or PGA.
3.4 Defects in Crystalline Solids
A perfect crystal is an idealization. Imperfections, often called defects, are present at random positions in the structure. These defects play an important role because they control several physico‐chemical properties of the solid. Metals, for example, would not show the attractive mechanical property of ductility when subjected to an applied stress were it not for the presence of a particular type of defect in the crystals called dislocations. Defects in crystals occur for two main reasons. Structurally, atoms in the pure material do not pack perfectly in a crystal due to thermal fluctuations during their processing or growth. Chemically, other atoms can be added accidentally or deliberately during production of the material, which disrupt the regular‐repeating pattern of the host atoms. As this type of compositional modification can influence the defect structure to a far larger extent than purely packing irregularities in the pure material, it is a widely used approach to modify or improve the properties of metals and ceramics.
Defects in crystalline solids are commonly divided into three types, described as point defects, line defects, and planar defects. Three‐dimensional defects, such as microcracks, pores, and fine particulate inclusions can be accidentally incorporated into a solid, whether crystalline or amorphous, during its production or use. These three‐dimensional defects have a significant effect on the bulk properties of a solid, such as its strength and the way it fractures under an applied mechanical stress ( Chapter 4).
3.4.1 Point Defects
Point defects are defects associated with one or a few atomic sites in the crystal. The major types of point defects are illustrated in Figure 3.16. A different atom, called a foreign atom, that occupies the site of a regular atom in the pure crystal, called a host atom, is said to be a substitutional defect. A vacant atomic site is called a vacancy. Foreign atoms and host atoms can occupy the interstitial sites between the regular atomic positions, giving foreign interstitial defects and self‐interstitial defects, respectively.
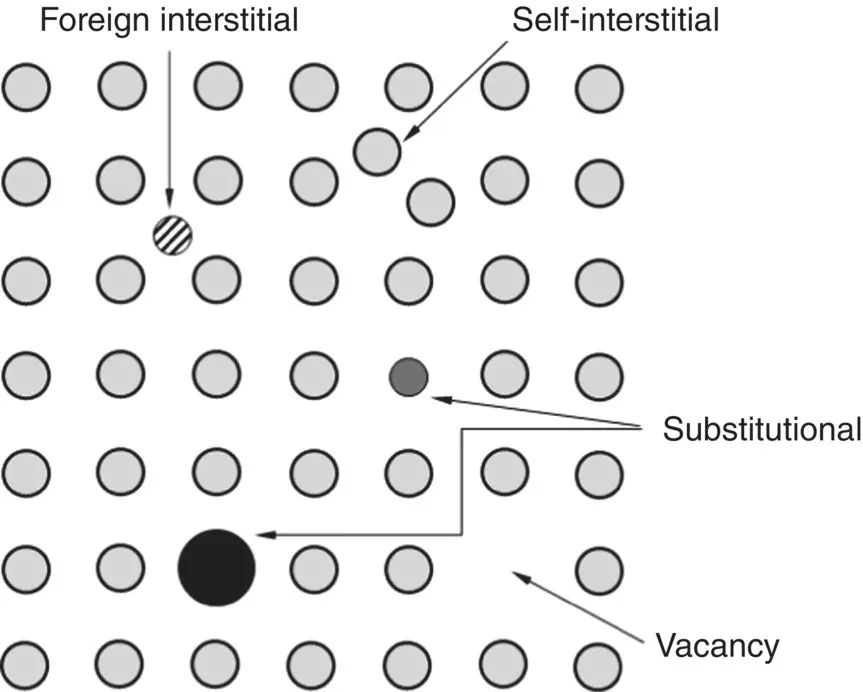
Figure 3.16 Schematic representation of the types of point defects in a crystal.
The majority of metals are not used in their pure state because they often have inadequate properties for most applications. Instead, other metals are commonly added to them in various amounts, forming alloys with an improved combination of properties such as higher strength, fatigue resistance, and corrosion resistance. Atoms of the alloying metal occupy sites in the host metal structure, forming substitutional or interstitial defects. The alloying metal is said to form a solid solution with the host metal. When examined at a scale larger than the atomic scale, a solid solution is homogeneous. It can be visualized as a solid‐state analog of a liquid solution such as a solution formed by dissolving common salt (sodium chloride) in water. The amount of one metal that can be alloyed with another metal to form a solid solution can vary from less than a fraction of a percent by weight (abbreviated wt%) to nearly 100 wt%, depending on the combination of metals. Copper, for example, can dissolve more than 30 wt% zinc to form brass, forming a substitutional solid solution in which the zinc atoms occupy the regular atomic sites of copper. On the other hand, iron can dissolve no more than ~0.007 wt% carbon at room temperature to form an interstitial solid solution known as mild streel. Brass and mild steel, however, are not suitable for use as biomaterials on account of their low corrosion resistance in vivo .
A Ti alloy that is widely used as implants in orthopedic and dental surgery is Ti6Al4V, composed of ~6 wt% aluminum and ~4 wt% vanadium (V), with the remainder (~90 wt%) being Ti ( Chapter 6). Although Ti6Al4V has an elastic modulus comparable to Ti, its superior strength, fatigue resistance, and corrosion resistance make it well suited for applications such as implants for total joint replacement, fracture fixation plates and dental implants. Certain stainless steels and cobalt–chromium (Co–Cr) alloys also find considerable use in orthopedic surgery.
Ceramic solid solutions are widely used in technological applications. One solid solution used in biomedical applications is YSZ, formed by adding ~3 mol% of yttria (Y 2O 3) to zirconia (ZrO 2). In this solid solution, the yttrium (Y) atoms predominantly occupy the regular zirconium (Zr) sites in the host ZrO 2structure, resulting in the formation of substitutional defects. Vacant defect sites (vacancies) are also created in the process to compensate for the difference in valence between Y and Zr.
The inorganic constituent of bone and teeth is not pure hydroxyapatite, Ca 10(PO 4)(OH) 2, but a highly substituted hydroxyapatite‐like material. This material has a structure that resembles that of hydroxyapatite but it contains approximately 4–8 wt% of (CO 3) 2−, often the main substituting species, smaller amounts of ions such as (HPO 4) 2−, Mg 2+and Na +(less than ~1 wt%), and trace amounts of ions such as Sr 2+, K +, F −, and Cl −(Posner and Betts 1975). While the defect chemistry of hydroxyapatite is complex, (CO 3) 2−and (HPO 4) 2−ions typically substitute at the anionic (PO 4) 3−sites in the crystal structure, whereas ions such Mg 2+, Na +, Sr 2+, and K +substitute at the cationic Ca 2+sites, and F −and Cl −ions substitute for OH −ions ( Figure 3.17).
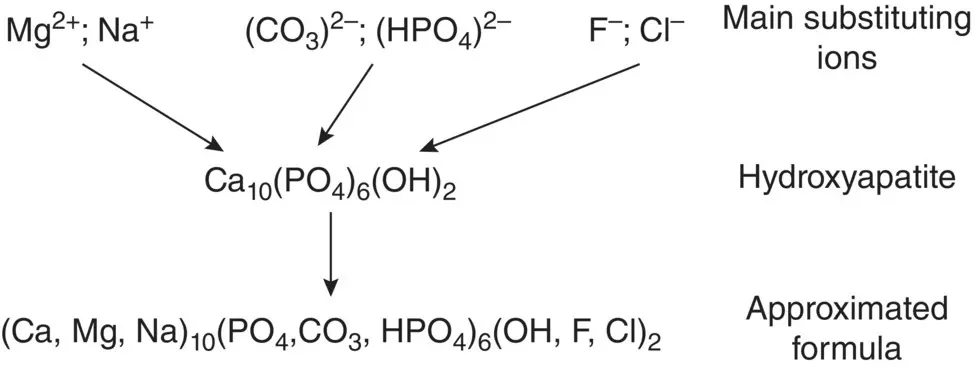
Figure 3.17 Major substituting ions and approximated formula of hydroxyapatite‐like constituent of bone.
3.4.2 Line Defects: Dislocations
A dislocation is a discontinuity in atomic packing that is associated with several atomic sites along a line in a crystal. The interatomic bonds are highly distorted in the immediate vicinity of a dislocation ( Figure 3.18), causing some degree of mechanical strain. Consequently, a crystal that contains dislocations has a higher energy than a similar but dislocation‐free crystal due to the energy associated with the mechanical strain. The higher the number of dislocations per unit volume, the higher the strain energy of the crystal. Because of the ease with which metal ions can break and reform bonds ( Chapter 2), the mechanical properties of metals are strongly dependent on the presence of dislocations. The property of ductility, for example, that makes metallic biomaterials such as Ti6Al4V, stainless steel and Co–Cr alloys attractive for use as fracture fixation plates, hip, and knee implants and dental implants is due to the presence of dislocations in these materials.
Читать дальшеИнтервал:
Закладка:
Похожие книги на «Materials for Biomedical Engineering»
Представляем Вашему вниманию похожие книги на «Materials for Biomedical Engineering» списком для выбора. Мы отобрали схожую по названию и смыслу литературу в надежде предоставить читателям больше вариантов отыскать новые, интересные, ещё непрочитанные произведения.
Обсуждение, отзывы о книге «Materials for Biomedical Engineering» и просто собственные мнения читателей. Оставьте ваши комментарии, напишите, что Вы думаете о произведении, его смысле или главных героях. Укажите что конкретно понравилось, а что нет, и почему Вы так считаете.