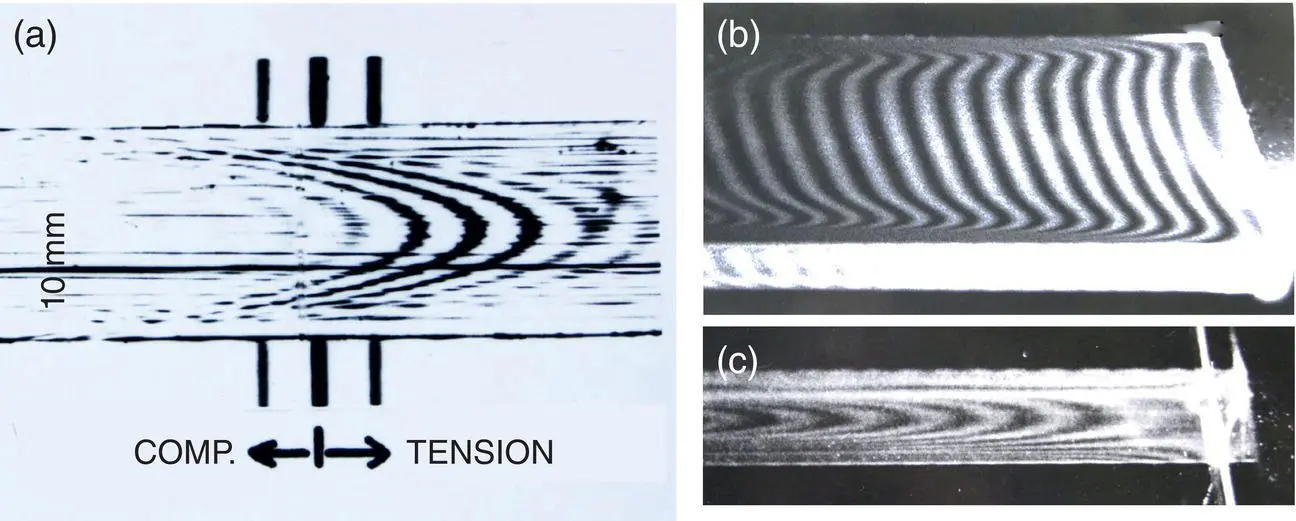
Figure 2.9 Thermally tempered lath with (a) parabolic stress distribution in white light through a Babinet compensator; (b) laser‐beam scattered‐light tomography revealing the stress distribution in the middle plane across width and (c) thickness.
Source: Sinha (1971).
In Section 4.11 of Chapter 4, we briefly describe the tempering of structural and automotive glass and its strength properties. The thermal tempering process involves heating the glass sheet uniformly to temperatures of around 650–700 °C, or measurably higher than the transformation range, T g, and then subjecting it to rapid cooling, usually by jets of air. Since cooling is usually symmetrical about the midplane of the glass plate, this process results in an approximately parabolic stress distribution in the glass plate with compression at the surfaces and tension in the midplane. It is recognized that the midplane tension, called “degree of temper,” is generally represented by the corresponding birefringence (double refraction). The depth‐dependent structural changes that occur in the glass plate during a toughening process induce this birefringence due to changes in the density and refractive indices within the plate, and planes parallel and perpendicular to the surfaces. The process of inducing small, but desirable, changes in the structure of the same type of optical glass by suitable heat treatment can also be applied in the fabrication of optical glass components.
2.4.5 Material Characteristics
As temperature rises, most materials, including glass, expand and density decreases. The coefficient of linear thermal expansion (CLTE), ε , is therefore expected to increase with increase in temperature. Figure 2.10illustrates this obtained from a slowly heated lath of window (soda‐lime–silica) glass. Since thermal history is important for glass, it is important to mention the heating rate(s) used in the experiment. The rate of increase of specimen temperature, T , was 2 °C/minute in the range 21–100 °C, 0.5 °C/minute in the range 100–400 °C, and about 0.25 °C/minute in the range of temperature above 400 °C. As expected, ε increased with increase in T , but the slope of the ε – T curve increased rapidly after about 500 °C. However, neither linearity below 500 °C nor an abrupt drop above this temperature was noticed. This contrasts with the observations of Lebedev (1926) on the temperature dependence of refractive index, presumably for a similar glass.
Figure 2.10 Temperature dependence of the CLTE for a lapped and polished lath (152.2 mm × 25.8 mm × 5.8 mm) taken from a large commercially annealed sheet of plate glass.
Source: Sinha (1971).
In addition to the temperature dependence of the refractive index of glass, one of the most important material characteristics is the dependence of elastic modulus on temperature. Figure 2.11shows the dependence of Young's modulus, E, of window glass on temperature. Unlike the sharp drop in refractive index, as mentioned earlier in Lebedev's studies, the value of E does not exhibit any drop between 500 and 600 °C, i.e. in the transition zone. However, E decreases monotonically with increase in temperature, but only slightly from its room temperature value. The commercially (as received) annealed glass exhibited a slightly lower value at temperatures less than 500 °C as compared to the laboratory annealed material. The difference indicates a thermal‐history‐related memory effect, similar to the thermal expansion coefficient of glass mentioned earlier. With increase in temperature and long soaking time during the mechanical testing for E, the commercially annealed glass could have approached structural equilibrium at temperatures higher than 500 °C.
Figure 2.11 Temperature dependence of Young's modulus, E, for commercially annealed and laboratory annealed automotive plate glass.
Source: Sinha (1971).
2.5 Rocks: The Most Abundant Natural Polycrystalline Material
Trinity of Rocks
SEDIMENTARY
METAMORPHIC
IGNEOUS
Rocks form due to the solidification of lava on Earth's surface and that of magma inside the earth. Lava refers to molten rock material that is extruded onto the surface during a volcanic eruption. Molten rock is known as magma when it lies below the ground surface. There are three basic types of rocks: sedimentary, metamorphic, and igneous; these types are briefly described in the following section.
We cannot define a rock as a solid in terms of a melting point. The melting point of rocks cannot be defined in a simplistic manner because they consist of different minerals with different melting points. In the field of engineering geology, a rock is defined not only in terms of the thermal state, but also as a hard solid that consists of compacted aggregation of minerals that remains intact in water and cannot be excavated without blasting (West 1995). In terms of T m, the definition of solid rock is, therefore, not as simplistic as one may think – as in polycrystalline pure metals, ice, and many ceramics.
The surface of Earth consists of about 75% sedimentary rocks. These rocks are recognized readily by the most noticeable feature of stratification or layers, often enriched with fossils. There is a wide range of sedimentary rocks depending upon the origin of their source material. West (1995) stated that 99% of all sedimentary rocks consist of shales (46%), sandstones (32%), and limestones (22%). The engineering properties of sedimentary rocks vary greatly, but these types of rocks are not used at high temperatures. Deep down in the earth, sedimentary rocks are subjected to metamorphic process due to heat and pressure.
Particles in the form of sand and silt produced from erosion of rocks are carried out by streams and rivers to the oceans or large lakes. The transported particles are consolidated by processes known as lithification. The Ancient Greek word “lithos” meaning rock and the suffix “ific” derived from Latin are combined to make “lithification” for describing the processes in which water‐saturated unconsolidated sediments compact under pressure to become “sedimentary rocks.” Lithification is therefore a time‐dependent process of the removal of fluid from pores through compaction and cementation. Millions of years of lithification processes of compaction, cementation, and crystallization of sediments deposited in water produce sedimentary rocks.
Lithification should not be confused with the word petrification that involves the replacement of organic material by silica over a long period of time, such as in the development of fossils (Monroe et al. 2006). However, there is a counterpart of the lithification processes in the case of the densification of snow or ice particles deposited on Earth's surface. In this case, air trapped in unconsolidated and porous snow deposits is removed under pressure and the mass undergo morphological changes by compaction, grain growth, and cementation (sintering). The primary difference is the extremely high thermal states of snow deposits. This subject will be covered later. Very similar processes are also involved in making ceramics and powder metallurgy.
Читать дальше