FIGURE 1.23 Comparison of energetics for the concerted and the enzyme (carbonic anhydrase) catalyzed stepwise reactions of H 2O and CO 2giving HCO 3 −.
Research by vibrational sum frequency (VSF) spectroscopy has shown that on the hydrophobic interface between water and an organic layer, such as CCl 4and hexane, the intermolecular hydrogen bonding between water molecules is substantially weaker than the hydrogen bonds of water in the liquid–vapor interface and inside the liquid phase of water. This is indicated by a blue shift of the vibrational bands for hydrogen bonded water in H 2O/CCl 4and H 2O/hexane interfaces relative to that for the water in the liquid–vapor interface [13]. This weakening in hydrogen bonding allows water molecules in the interface to move more freely and gives rise to strong orientation effects of water on the hydrophobic interface, such that the O─H bond in some water molecules (1 in 4 H 2O molecules) on the hydrophobic interface penetrates into the organic layer [9, 14]. In the cases of the heterogeneous organic reactions performed in water, if a reactant molecule can act as a hydrogen bond acceptor, the OH bond in water which penetrates into the organic layer can form a strong hydrogen bond with the reactant molecule and more importantly, form a strong hydrogen bond with the transition state of the reaction to stabilize the transition state and lower the activation energy[9, 14]. In addition, upon vigorous agitation of the reaction mixtures in water, the bulk organic phase can be separated into aggregates (droplets) by water. Formation of aggregates increases surface area of the reactants and therefore, enhances their energy to lower the activation energy as well [9]. As a result, the reactions that take place on the hydrophobic interface (heterogeneous reactions) become much faster than the homogenous reactions occurring in organic solvents or in neat reactants [9, 14]. The hydrophobic effects are summarized in Figure 1.24. This way water is not only used as a green solvent, but it also acts as an effective “catalyst” to speed up the reactions.
Figure 1.24a illustrates that a free OH bond in a water molecule of the hydrophobic interface partially penetrates into the organic layer and is hydrogen bonded to the transition state (via a hydrogen bond acceptor) of a reaction taking place in the organic phase. This water molecule is also hydrogen bonded to other water molecules (denoted by W) inside the water phase. The hydrogen in the free OH bond acts as a nearly bare proton. Therefore, the hydrogen bond formed on the transition state is strong, greatly stabilizing the transition state ( Fig. 1.24b). [TS] O ǂand [TS] W ǂrepresent the transition states for the reactions taking place in organic media (homogeneous) and in hydrophobic water/organic interface (heterogeneous), respectively. E a,Oand E a,Ware the corresponding activation energies for the homogeneous and heterogeneous reactions ( E a,W< E a,O), respectively. Both aggregation of the organic reactants and especially, the hydrogen‐bond stabilization for the transition state result in decrease in the activation energies of the organic reactions on the hydrophobic interface and greatly speed up the reactions relative to those performed in organic media.
FIGURE 1.24 Hydrophobic effects on organic reactions. (a) The intermolecular hydrogen bond between water and transition state of reactions taking place in the hydrophobic interface, and (b) comparison of the energetics for the homogeneous reactions ( E a,Oand [TS] O ǂ) in organic media and heterogeneous reactions ( E a,Wand [TS] W ǂ) in the water–organic interface.
Many types of organic reactions, such as Diels–Alder cycloadditions and Claisen rearrangements, have been carried out in water with fast speeds and good yields [9–12]:
(1.74) 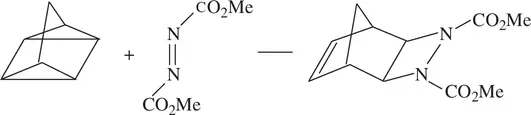
For example, it only takes 10 min for Reaction 1.74to complete in water as the reaction mixture is vigorously agitated at 23 °C. When the reaction is performed by neat reactants with no solvent, the completion time is 48 h. It takes more than 120 h for the same reaction to complete in toluene homogeneously [12].
(1.75) 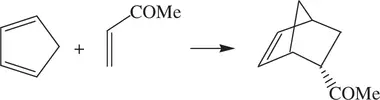
The rate constant for Reaction 1.75performed in water ( k water) is many times greater than the rate constants for the reaction performed in organic solvents ( k solvent). Research has shown that the k water/ k solventratios at room temperature for isooctane, acetonitrile, and methanol are 740, 290, and 58, respectively [9].
More examples and mechanistic details of organic reactions carried out in water will be discussed in individual chapters ( Chapters 4, 8, and 10) in the sections of green chemistry methods and applications.
1 1.1 Dibenzylmercury (PhCH2)2Hg contains linear C─Hg─C bonds. At 140 °C the compound undergoes thermal decomposition to give 1,2‐diphenylethane PhCH2CH2Ph and mercury. Suggest a concerted mechanism and a stepwise mechanism for this reaction.
2 1.2 The following are two alternative mechanisms for the AlCl3 catalyzed Friedel–Crafts reaction of benzene with chloroethane. Show qualitative reaction profiles for both mechanisms. Which mechanism is more plausible? Explain.
3 1.3 The decomposition of bromoethane in gaseous phase to ethene and hydrogen bromide follows a first‐order rate law. Suggest a possible concerted and a stepwise mechanisms which are consistent with the rate law.
4 1.4 The rate of nitration of benzene in a mixture of nitric acid and sulfuric acid does not depend on the concentration of benzene. What conclusion can be drawn from this? Show qualitative energy profile for the stepwise reaction.
5 1.5 The mechanism for the autoxidation of hydrocarbons by oxygen is shown below:Apply the steady‐state approximation to work out the expected kinetics for the reaction.
6 1.6 For the SN1 solvolysis of the tertiary chloroalkanes R–Cl in ethanol at 25°C, the relative rates for three different chloroalkanes are 1, 1.2, and 18.4 for R = Me3C, tBuMe2C, and tBu2MeC, respectively. Is the difference due to electronic or steric effects? Explain. Draw qualitative energy profiles for the SN1 solvolysis of the three chloroalkanes.
7 1.7 For the following each pair of anions, compare their basicity and nucleophilicity. Account for the difference.(1) OH− and SH− (2) CH3COO− and C6H5COO−
8 1.8 Which of the following SN2 reactions goes faster? Explain by kinetic isotope effect.
1 1. Jackson, R. A. Mechanisms in Organic Reactions, The Royal Society of Chemistry, Cambridge, UK (2004).
2 2. Silbey, R. J.; Alterty, R. A.; Bawendi, M. G. Physical Chemistry, 4th ed., Wiley, Danvers, MA, USA (2005).
3 3. Hoffman, R. V. Organic Chemistry: An Intermediate Text, 2nd ed., Wiley, Hoboken, NJ, USA (2004).
4 4. Huheey, J. E.; Keiter, E. A.; Keiter, R. L. Inorganic Chemistry: Principles of Structure and Reactivity, 4th ed., Harper Collins, New York, NY, USA (1993).
Читать дальше