Very often, isotope labeling on a compound may alter the rate constants for certain reactions of the compound. This is referred to as the kinetic isotope effects[1, 3]. In principle, a heavier isotope of an element has a lower zero‐point energy. Therefore, a bond to a heavier isotope (such as C─D bond) possesses a lower energy level than a bond to a lighter isotope (such as C─H bond) ( Fig. 1.19). More energy is required to break a bond to a heavier isotope (such as C─D bond) than a bond to a lighter isotope (such as C─H bond). Since breaking of a C─H/C─D bond has a late transition state, the difference in energies of the transition states for breaking these bonds is much smaller than the difference in energy levels of the C─H and C─D bonds. Thus, the activation energy for breaking a C─D bond ( E a,D) is greater than that for breaking a C─H bond ( E a,H) ( Fig. 1.19). This means the activation energy for a reaction that involves breaking a bond formed on a heavier isotope (such as C─D bond) is greater, corresponding to a smaller rate constant at a given temperature, if ( only if ) breaking of the bond is the rate‐determining step for the studied reaction. If breaking of this bond occurs prior to or after the rate‐determining step, the kinetic isotope effect is very minor. This effect is most remarkable for substitution of deuterium (D) for hydrogen (H) because the ratio in masses between these two isotopes (D/H = 2) is larger than those for any other pairs of isotopes. In general, if a bond to hydrogen (such as C–H) or deuterium (such as C–D) is being broken in the rate‐determining step of a reaction, the ratio of k H (rate constant for the reaction involving breaking the bond to hydrogen, such as C─H bond) to k D (rate constant for the reaction involving breaking the bond to deuterium, such C─D bond), k H /k D , is typically 2–8 . This is termed kinetic deuterium isotope effect. If the k H/ k Dvalues are found between 1 and 1.5, the corresponding bond breaking does not occur in the rate‐determining step.
FIGURE 1.18 Reaction mechanism for acid‐catalyzed hydrolysis of the oxygen‐18 isotope labeled ethyl acetate.
FIGURE 1.19 Energetics for C─H and C─D (deuterium) bonds.
Kinetic deuterium isotope effect is often used in studies of the C─H bond functionalization. For example, the radical bromination of toluene (PhCH 3) to benzyl bromide (PhCH 2Br) has the kinetic isotope effect k H/ k D~ 5 [3]. This shows that breaking of the C─H bond in the methyl group of toluene by a bromine radical ( Reaction 1.71) is the rate‐determining step for the overall bromination reaction:
(1.71) 
The mechanism of radical halogenations of alkanes will be discussed extensively in Chapter 2.
The electrophilic nitration of benzene by acetyl nitrate (CH 3CO 2NO 2) involves substitution of the aromatic C─H bond by a nitro (–NO 2) group ( Reaction 1.72) [3]:
(1.72) 
Overall, the reaction has the second‐order rate law, first order in benzene and first order in acetyl nitrate (rate = k [C 6H 6][CH 3CO 2NO 2]). Study of the kinetic isotope effect using fully deuterated benzene C 6D 6shows k H/ k D= 1 [3]. The data indicate that the C─H bond cleavage is not involved in the rate‐determining step. Instead, the rate‐determining step is the electrophilic attack of the nitrating agent on benzene, which is supported by the observed overall second‐order rate law. Mechanism for the reaction is shown in Figure 1.20. The aromatic nitration and related electrophilic substitution reactions of arenes will be studied in Chapter 5.
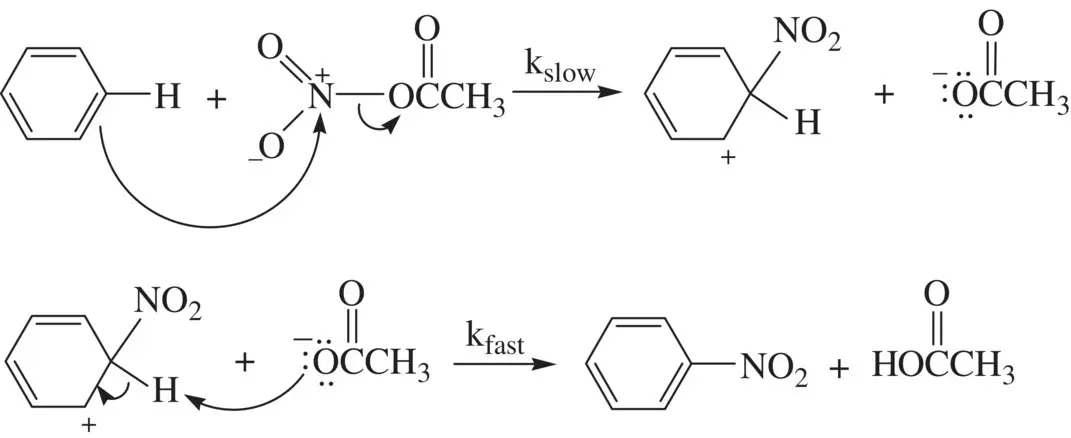
FIGURE 1.20 Reaction mechanism for nitration of benzene by acetyl nitrate.
1.11 ENZYMES: BIOLOGICAL CATALYSTS
Biochemistry is the study of life at the molecular level. Among main types of biomolecules are carbohydrates (including monosaccharides, disaccharides, and polysaccharides), lipids (including triacylglycerols, phospholipids, and steroids), proteins, and nucleotides (e.g., ATP) and nucleic acids (including RNA and DNA). Most of the biochemical reactions that occur in vivo (in the living systems such as animals and plants) are catalyzed by enzymes, which are also called biological catalysts. Almost all the enzymes are protein molecules which are composed of α‐amino acid residues linked by peptide bonds. There are six types of enzymes classified on the basis of the types of reactions that the enzymes catalyze. They are oxidoreductases (the enzymes catalyzing oxidation–reduction reactions), transferases (the enzymes catalyzing group transfers, namely to substitute one functional group for another), lyases (the enzymes catalyzing elimination of two groups from adjacent carbon atoms to form a C=C double bond), hydrolases (the enzymes catalyzing hydrolysis of biomolecules), isomerases (the enzymes catalyzing isomerization reactions), and ligases (the enzymes catalyzing bond formation coupled with ATP hydrolysis) [8].
An enzyme contains an active sitewhich is the catalytic center where a biochemical reaction takes place. The active site is usually composed of functional groups from side chains of some α‐amino acid residues in the protein (enzyme) molecule. The biological reactant molecule(s) [often termed substrate(s), one or more] enters the structure of the enzyme and loosely binds to the enzyme usually by hydrogen bonding, hydrophobic interactions, and/or ionic bonding to form an intermediate enzyme–substrate complex(ES complex). Then various chemical processes take place in the ES complex to lead to the formation of the product. Finally, the product departs from structure of the enzyme to regenerate a free enzyme molecule. The enzyme catalyzed biochemical reactions are generalized as follows ( Eq. 1.73):
(1.73) 
In Equation 1.73, S and E represent the biological substrate and enzyme, respectively. ES is the intermediate enzyme–substrate complex. P is the reaction product. The formation of the ES complex is usually reversible, while the conversion of ES to the final product is irreversible.
An enzyme catalyzes a biochemical reaction by lowering activation energy of the reaction. On the basis of the types of interactions between the enzyme and the biological substrate in the transition state of a biochemical reaction, there are in general three types of catalytic mechanisms.
Читать дальше