The neutral haloalkane molecules RX (X = Cl, Br, or I) function as another common type of electrophiles owing to polarity of the carbon–halogen bond. The slightly positively charged carbon in the carbon–halogen bond of a primary or secondary haloalkane can be attacked by a strong nucleophile (usually, an anion) effecting an S N2 reaction ( Reaction 1.66).
(1.66) 
A carbonyl group (C=O) functions as an electrophile, with the reactive (electrophilic) center being the slightly positively charged carbon atom as described earlier in Section 1.8.3( Fig. 1.13). As a result, a ketone (or an aldehyde) can undergo a nucleophilic addition with a strong nucleophile as shown in Reaction 1.67:
(1.67) 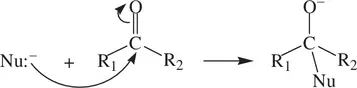
A Bronsted (protic) acid (a proton donor) can always accept a pair of electrons from nucleophiles via the proton attached to the acid molecule. Therefore, the Bronsted acid is also a Lewis acid or an electrophile. For example, a hydrogen halide (H–X) molecule functions as an electrophile in Reaction 1.64, with the reactive center being the electrophilic proton. In addition, a protic acid can protonate the carbonyl oxygen, which is nucleophilic because of the lone pairs of electrons ( Reaction 1.68):
(1.67) 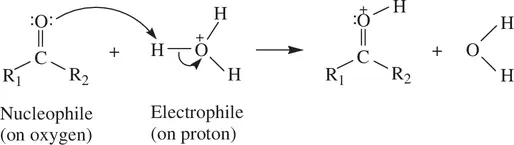
Some strong Lewis acids such as AlCl 3, BF 3, and BH 3(generated by dissociation of B 2H 6) are also strong electrophiles commonly used in many organic reactions. The electrophilicity of these species lies in the active empty p orbitals in the central aluminum and boron atoms. The functions of the compounds will be discussed extensively in Chapters 3and 5.
1.9.2 Common Nucleophiles
Many anions that contain a lone pair of electrons (e.g., OH −, OR −, RCO 2 −, HS −, Br −, and CN −) are good (strong) nucleophiles. On the other hand, many electrically neutral molecules that contain a lone pair of electrons (e.g., H 2O, ROH, and RCO 2H) act as poor (weak) nucleophiles.
When we determine the relative strength of nucleophiles, consideration is focused on the reactivity for the nucleophiles toward electrophilic carbon atoms of organic substrates. The general guideline is The greater the electron density does the species have on its reactive center and the larger is the size of the reactive center, the more nucleophilic is the species . On the basis of this guideline, we have the following general rules:
1 An anion is stronger in nucleophilicity than a neutral molecule given that the reactive (nucleophilic) centers for both the anion and neutral molecule are the atoms of the same element. This is because in general an anion, due to its negative charge, has greater electron density than does a neutral molecule. It can be readily seen by comparison of hydroxide (OH−, a strong nucleophile) and water (H2O, a weak nucleophile) and by comparison of an alkoxide (RO−, a strong nucleophile) and an alcohol (ROH, a weak nucleophile).For both pairs of species, the reactive centers are in oxygen atoms. The above Lewis structures show that each of the anions has three lone pairs of electrons in the oxygen atom, while the corresponding neutral molecule has two lone pairs of electrons in the oxygen atom. Clearly, for each pair of the species, the anion has greater electron density in the reactive center than the corresponding neutral molecule, giving rise to stronger nucleophilicity.
2 For the same group of elements, the atomic radii increase from the top to bottom. As a result, for species containing the atoms of the same group of elements as reactive centers and having the same number of electron pairs in the reactive centers, their nucleophilicity increases as one moves from the top to the bottom along the group of elements. For example, the nucleophilicity of halides increases in the order of fluoride (F−), chloride (Cl−), bromide (Br−), and iodide (I−). All of them have four electron pairs. Another example is that the nucleophilicity of hydrogen sulfide (SH−) is stronger than hydroxide (OH−). For both of them, the central atoms contain three lone pairs of electrons.
Some molecules do not contain any lone pairs of electrons. However, a bonding electron pair in these molecules may be donated to an electrophile making them nucleophilic. In this category, compounds containing a C–M [M = Li or MgX (X = Cl, Br, or I)] bond are strong nucleophiles because the C─M bonding electron pair is active and has strong tendency to be donated to an electrophilic center. For example, a Grignard reagent undergoes nucleophilic addition to a carbonyl group (a carbon electrophile) as shown below:
The C=C π bond in alkenes is nucleophilic. They are characterized by electrophilic addition reactions (e.g., Reaction 1.64). The aromatic rings are nucleophilic due to the activity of the conjugate π electrons. Therefore, arenes undergo extensive electrophilic substitution reactions as illustrated below:

The enrichment of specific isotope of an element for an atom in a compound molecule is called isotope labeling. It is a very useful technique for studying kinetics and mechanisms for organic reactions. For example, let us first consider the acid‐catalyzed hydrolysis of esters such as ethyl acetate ( Reaction 1.69) [1]
(1.69) 
From the overall reaction, it is not clear what bond, the acyl‐oxygen bond or the alkyl‐oxygen bond, is broken. Cleavage of either bond could lead to the formation of the products.
In order to establish the mechanism for the reaction, the oxygen atom of the alkoxide group (–OEt) in the ester is labeled (enriched) with the oxygen‐18 isotope ( 18O) and the hydrolysis of the 18O‐labeld ester is conducted in the same condition ( Reaction 1.70):
(1.70) 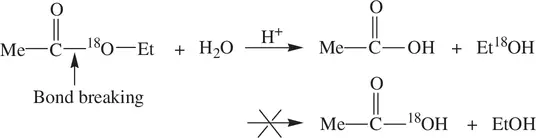
Et 18OH is identified from the products by mass spectrometry, but EtOH is not found. MeCO 18OH is not found either [1]. The results unambiguously show that the acyl‐oxygen bond is broken in the reaction giving the observed products. However, the alkyl‐oxygen bond remains intact, as cleavage of the alkyl‐oxygen bond would lead to MeCO 18OH, but it is not observed. On the basis of the oxygen‐18 isotope labeling experiment and other supporting data, the reaction mechanism is established and described in Figure 1.18. The acyl–oxygen bond cleavage, unambiguously identified from the isotope labeling experiment, is accomplished via the combination of a nucleophilic addition of water and a subsequent elimination of Et 18OH.
Читать дальше