Applied Water Science
Здесь есть возможность читать онлайн «Applied Water Science» — ознакомительный отрывок электронной книги совершенно бесплатно, а после прочтения отрывка купить полную версию. В некоторых случаях можно слушать аудио, скачать через торрент в формате fb2 и присутствует краткое содержание. Жанр: unrecognised, на английском языке. Описание произведения, (предисловие) а так же отзывы посетителей доступны на портале библиотеки ЛибКат.
- Название:Applied Water Science
- Автор:
- Жанр:
- Год:неизвестен
- ISBN:нет данных
- Рейтинг книги:4 / 5. Голосов: 1
-
Избранное:Добавить в избранное
- Отзывы:
-
Ваша оценка:
- 80
- 1
- 2
- 3
- 4
- 5
Applied Water Science: краткое содержание, описание и аннотация
Предлагаем к чтению аннотацию, описание, краткое содержание или предисловие (зависит от того, что написал сам автор книги «Applied Water Science»). Если вы не нашли необходимую информацию о книге — напишите в комментариях, мы постараемся отыскать её.
This book explores the basic concepts and applications of water science. It provides an in-depth look at water pollutants’ classification, water recycling, qualitative and quantitative analysis, and efficient wastewater treatment methodologies. It also provides occurrence, human health risk assessment, strategies for removal of radionuclides and pharmaceuticals in aquatic systems. The book chapters are written by leading researchers throughout the world. This book is an invaluable guide to students, professors, scientists and R&D industrial specialists working in the field of environmental science, geoscience, water science, physics and chemistry.
Applied Water Science — читать онлайн ознакомительный отрывок
Ниже представлен текст книги, разбитый по страницам. Система сохранения места последней прочитанной страницы, позволяет с удобством читать онлайн бесплатно книгу «Applied Water Science», без необходимости каждый раз заново искать на чём Вы остановились. Поставьте закладку, и сможете в любой момент перейти на страницу, на которой закончили чтение.
Интервал:
Закладка:

Figure 1.1The chemical structures of PAEs. Adapted from [1]. PAEs, phthalic acid esters.
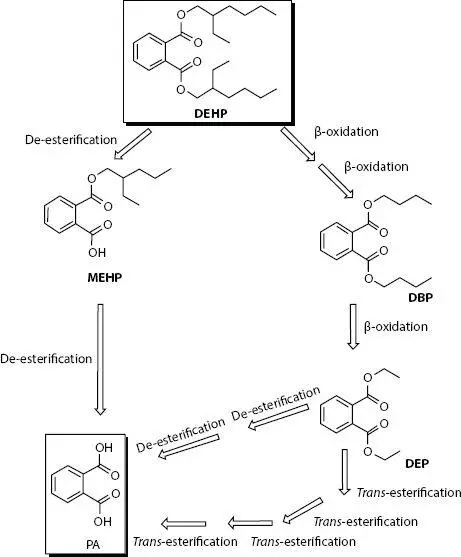
Figure 1.2DEHP biodegradation pathways to obtain MEHP, DBP, and DEP. Reprinted from [14] with permission from Elsevier. DBP, dibutyl phthalate; DEHP, di-2-ethylhexyl phthalate; DEP, diethyl phthalate; MEHP, mono-2-ethylhexyl phthalate; PA, polyacrylate.
PAEs have been analyzed in water samples using gas chromatography (GC) coupled to flame ionization detectors (FIDs) [20], mass spectrometry (MS) [21] and tandem MS (MS/MS) [22], or highperformance liquid chromatography (HPLC) coupled to diode array detectors (DADs) [23], ultraviolet (UV) [24], and MS [25]. Among them, GC is normally the preferred technique since most PAEs are nonpolar and thermostable. It is important to notice that, in all these analytical methods, it has been necessary to include previous sample preparation steps before instrumental analysis to achieve accurate and sensitive results. These steps consist on the isolation and pre-concentration of PAEs since they can be found in water samples at extremely low concentrations. However, since PAEs are not ionizable in water, these samples are normally analyzed directly or after a simple filtration without pH adjustment regardless of the sample preparation technique used in each case [26].
In this context, special attention should be paid to the risk of sample contamination during their analysis, which would result in false positives and/or over-estimated concentrations. As it has already been said, PAEs are ubiquitous contaminants and this includes their possible presence in any laboratory since they can be found in solvents, reagents, filters, etc. Consequently, previous washing steps using PAE-free solvents, if possible (since most organic solvents also contain some PAEs), subsequent heating of non-volumetric glassware at high temperatures (450–550°C) for several hours (4–5 h), washing volumetric or any glassware material with strong oxidizing agents, and, in some cases, even wrapping in heat-treated aluminum foil to avoid adsorption of PAEs from the air are carried out, among others [27–29]. Despite all these precautions, residues of PAEs may finally appear, and the analysis of blanks should be developed on a daily basis in every batch of samples so that background levels can be suitably subtracted [21, 25, 30].
Until very recently, the most widely used sample preparation methods, also for the analysis of PAEs in water samples, have been based on the use of liquid-liquid extraction (LLE) and solid-phase extraction (SPE) [31, 32]. The need for developing quicker, simpler, and miniaturized extraction procedures able to maintain or even to improve the required sensitivity of the analysis has resulted in the development of new sample preparation techniques. In this sense, microextraction techniques have gained notoriety since the extraction is carried out using amounts of extracting phase much smaller than the sample amount (extraction of analytes is not always exhaustive). Microextraction techniques have inherent advantages such as exceptionally high enrichment factors, simplicity, time saving, and the generation of small amounts of organic solvent or reagents wastes, without affecting reproducibility, and compatibility with most analytical instrumentation [33–36]. Among these new alternatives, sorbent-based microextraction techniques have been widely used due to the great diversity of commercially available sorbents, as well as new extraction sorbents (in particular nanomaterials) that are constantly being proposed for their direct use or after a previous functionalization to enhance their selectivity [35–37].
As a result of the above-mentioned issues, the aim of this book chapter is to provide a general overview of the sorbent-based microextraction techniques applied to the analysis of PAEs in water samples, which mainly include solid-phase microextraction (SPME), dispersive SPE (dSPE), and magnetic dSPE (m-dSPE), among others. The extraction ability to quantitatively and selectively extract these target analytes will be commented and discussed.
1.2 Solid-Phase Microextraction
SPME has been the sorbent-based microextraction technique most used for the analysis of PAEs in water samples (see Table 1.1) probably, among other reasons, because it allows to reduce the risk of PAEs contamination during sample extraction with respect to other conventional extraction techniques. On the one hand, the absence of organic solvents and additional steps reduces PAEs background levels. On the other, water is in many occasions a simple and clean matrix that contains few interferences, so the direct immersion (DI) mode can be used without hardly any impairment of its lifetime (except for waste waters or marine water). Moreover, in SPME, extraction, pre-concentration and direct desorption into analytical instruments can be easily integrated in most cases.
The first studies in which SPME was applied for PAEs extraction from water samples dealt with the direct application of commercial fiber coatings, including polydimethylsiloxane (PDMS), polyacrylate (PA), PDMS-divinylbenzene (DVB), carboxen (CAR)-PDMS, and carbowax (CW)-DVB. As examples, Cao [21] demonstrated the better performance of PDMS-DVB fibers compared to PDMS and DVB-CAR-PDMS fibers for the headspace (HS) SPME extraction of nine PAEs (DMP, DEP, DIBP, DBP, BBP, DHXP, DEHA, DEHP, and DNOP) from bottled water samples, while Polo et al. [28] found that PDMS-DVB fibers also give higher extraction efficiency than PDMS, PA, CAR-PDMS, and CW-DVB fibers for DBP, BBP, and DNOP, but CAR-PDMS and PA fibers show a better extraction performance for DMP and DEP, and for DEHP, although the first one provided better results for simultaneous analysis of the target PAEs from bottled, industrial harbor, river, urban collector, and influent and effluent waste water samples. As expected, the optimal SPME fiber for the extraction of a particular phthalate depends on both the properties of the coating and the PAEs since these compounds differ from each other in terms of polarity and volatility and, therefore, on their distribution between the fiber coating and the matrix. In addition, low-molecular PAEs are more volatile than those of high-molecular weight [38]. As a result, low-molecular PAEs would be expected to be more efficiently extracted when HS mode is used [38]. However, they have a certain solubility in water and, as consequence, they volatilize very slowly from this kind of matrices. Contrary, although high-molecular PAEs are less volatile, they have a lower water solubility and they volatilize faster at higher temperatures than it could be expected [38]. Accordingly, it has been observed that DEHP and DNOP are extracted from different water samples more efficiently than BBP, DEP, and DMP using HS-SPME [28, 39]. Nevertheless, most of the works published on this topic are based on DI-SPME instead of HS-SPME.
Table 1.1Some examples of the application of SPME and SBSE for the analysis of PAEs in water samples.
PAEs | Matrix (sample amount) | Sample pretreatment | Separation technique | LOQ | Recovery study | Residues found | Comments | Reference |
SPME | ||||||||
DMP, DEP, DBP, BBP, DEHP, and DNOP | Mineral, river, industrial port, sewage, and waste waters (10 mL) | SPME using a PDMS-DVB fiber, stirring at 100°C in DI mode for 20 min, and desorption at 270°C for 5 min | GC-MS | 0.0067–0.34 μg/L | 87–110% at 0.5 and 2.5 μg/L | One sample of each water were analyzed and contained all PAEs at levels from 0.011 to 6.17 μg/L | A multifactor categorical design was used for optimization purposes. PDMS-DVB fiber showed higher extraction efficiency than PDMS, PA, CAR-PDMS and CW-DVB fibers for DBP, BBP, and DNOP, but CAR-PDMS for DMP and DEP, and PA for DEHP. DI-SPME provided better sensitivity than HS mode | [28] |
DEHA, DMP, DEP, BBP, DIBP, DBP, DHXP, DEHP, and DNOP | Mineral water (10 mL plus 10 or 30% w/v NaCl) | SPME using a PDMS-DVB fiber, stirring at 90°C in DI mode for 60 min, and desorption at 270–280°C for 5 min | GC-MS | - | - | Eleven samples were analyzed and residues of DEP, DIBP, DBP, and DEHP were found at levels from 0.052 to 1.72 μg/L | PDMS-DVB fiber showed higher extraction efficiency than PDMS and DVB-CAR-PDMS fibers | [21] |
DPP, DBP, DIBP, and DNPP | Mineral and tap water (10 mL) | SPME using a MWCNTs-PPy fiber, stirring at room temperature in DI mode for 60 min, and desorption at 250°C for 25 min | GC-FID | 0.17–0.33 μg/L | 90–113% at 5 and 50 μg/L | Three mineral water samples and 1 tap water were analyzed and contained at least 1 PAE at levels from 0.6 to 7.90 μg/L, except 1 of the mineral water samples | - | [40] |
DMP, DEP, DBP, DAP, and DNOP | Mineral, tap and reservoir waters (12 mL plus 10% w/v NaCl) | SPME using a MIP fiber, stirring at 60°C in DI mode for 30 min, and desorption at 250°C for 10 min | GC-MS | 0.0072–0.069 μg/L | 94.54–105.34% | One sample of each water were analyzed and contained at least 2 PAEs at levels from 0.07 to 0.53 μg/L | DBP was used as the template molecule. MIP fiber showed higher extraction efficiency than a non-imprinted polymer fiber, and PDMS, PA and CW-DVB fibers | [45] |
DMP, DEP, DBP, BBP, DEHP, DINP, and DNOP | Water (5 mL plus 6% w/v NaCl) | SPME using a PA fiber, stirring at room temperature in DI mode for 50 min, and desorption at 270°C for 2 min | GC-MS | 0.007–0.027 μg/L | - | Six samples were analyzed and contained at least 2 PAEs at levels from 0.4 to 78.8 μg/L | PA fiber showed higher extraction efficiency than PDMS fiber. Urine was also analyzed | [95] |
DIBP, DBP, BMPP, DNPP, DHXP, BBP, DCHP, DEHP, DIPP, DNOP, and DINP | River and tap waters (- mL) | SPME using a bamboo charcoal fiber, stirring at room temperature in DI mode for 20 min, and desorption at 280°C for 10 min | GC-MS | 0.013–0.067 μg/L | 61.9–87.1% at 0.1, 0.5, and 1 μg/L | One sample of each water were analyzed and no residues were detected | Bamboo charcoal fiber showed greater extraction efficiency than PDMS, PDMS-DVB and PA fibers for DNOP and DINP, but lower for DIBP, DBP, and DNPP | [47] |
DBP, DIBP, BBP, and DEHP | Mineral water (9 mL plus 20% w/v NaCl) | SPME using a TiO 2NPs fiber, stirring at 30°C in DI mode for 75 min, and desorption at 285°C for 5 min | GC-FID, GC-MS | 0.17–0.40 μg/L | 86–107% at 2μg/L | One sample was analyzed and residues of DIBP and DEHP were found at 1.0 and 2.2 μg/L, respectively | TiO 2NPs fiber showed better extraction efficiency than PDMS and poly(3,4-ethylenedioxythiophene)-TiO 2fibers. DI-SPME provided better sensitivity than HS mode | [39] |
DMP, DEP, DBP, and DEHP | Mineral, river and tap waters (15 mL) | SPME using a SiO 2-PDMS-MWCNTs fiber, stirring at 40°C in DI mode for 30 min, and desorption at 280°C for 2 min | GC-FID | 0.033–0.067 μg/L | 79.62–109.3% at 10 μg/L | One sample of each water were analyzed and residues of DBP and DEHP were found at 5.26 and 8.47 μg/L, respectively, in the mineral water sample | SiO 2-PDMS-MWCNTs fiber showed better extraction efficiency than PDMS, PA and DVB-CAR-PDMS fibers | [43] |
DPP, DIBP, DBP, DNPP, BBP, and DEHP | Mineral and tap waters (10 mL) | SPME using a poly- o -aminophenol-MWCNTs fiber, stirring at 35°C in DI mode for 60 min, and desorption at 280°C for 2 min | GC-FID | 0.10–0.25 μg/L | 91–115% at 5 and 50 μg/L | Three mineral water samples and 1 tap water sample were analyzed and contained at least 2 PAEs at levels from 0.3 ± 0.02 to 8.1 ± 0.19 μg/L, except for 1 mineral water sample | NaCl and dextrose injection solutions were also analyzed | [42] |
DBP, BBP, DEHA, DEHP, and DNOP | Tap, barreled drinking and pond waters (10 mL plus 15% w/v NaCl) | SPME using a PS-MWCNTs fiber, stirring at room temperature in DI mode for 60 min, and desorption at 280°C for 5 min | GC-MS/MS | 0.0038-0.059 μg/L | 73.4-103.8% at 0.05 and 0.2 μg/L | One sample of each water were analyzed and contained at least 1 PAE at levels from 0.038 ± 0.004 to 0.060 ± 0.007 μg/L | A Box-Behnken design was used for optimization purposes | [41] |
DPP, DBP, DEHA, and DEHP | Mineral, tanked and tap waters, and boiling water exposed to a PET container (10 mL plus 30% w/v NaCl) | SPME using a G-PVC fiber, stirring at 70°C in HS mode for 35 min, and desorption at 230°C for 4 min | GC-FID | 0.2–0.3 μg/L | 88–108% at 10 and 20 μg/L | One sample of each water were analyzed and residues of DPP and DBP were found at 2.1 and 1.8 μg/L, respectively, only in the boiling water exposed to a PET container | A central composite design was used for optimization purposes. Sunflower and olive oils were also analyzed | [20] |
DMP, DEP, DIBP, DBP, DMEP, BMPP, DEEP, DNPP, BBP, DHXP, DBEP, DCHP, DPhP, DEHP, DNOP, and DINP | Sea water (10 mL) | SPME using a PDMS fiber, stirring at 35°C in DI mode for 40 min, and desorption at 40°C for 6 min | GC-MS | 0.00017–0.0011 μg/L | 68.0–114.0%, but 55.4% for DMP, at 100 and 300 μg/L | Eleven sample was analyzed and contained at least 9 PAEs at levels from 0.270 to 1.39 μg/L | Sediment was also analyzed by conventional SPE | [96] |
DEP, DIBP, DBP, BBP, and DEHP | River, bottled and mineral waters (4 mL plus 20% w/v NaCl) | SPME using a polyamide6-MnO fiber, stirring at 80°C in HS mode for 30 min, and desorption at 200°C for 5 min | GC-μ-ECD | 0.13–0.64 μg/L | 90.3–106% at 10 and 100 μg/L | One sample of each water were analyzed and residues of DEP, DIBP and DBP were found at levels from 9.24 to 29.3 μg/L, respectively, in the bottle and mineral waters | Polyamide6-MnO fiber showed better extraction efficiency than PDMS fiber. Soda was also analyzed | [44] |
DMP, DEHP, DBP, DNPP, BBP, and DNOP | Tap and sea water (20 mL adjusted at pH 4) | SPME using a GO-1-(3-aminopropyl)-3-vinyl imidazolium bromide/tetrafluoroborate fiber, stirring at 35°C in DI mode for 30 min, and desorption at 175°C for 5 min | GC-MS | 0.017–0.10 μg/L | 87.6–101.2% at 1 and 5 μg/L | One sample of each water were analyzed, and no residues were detected | GO-1-(3-aminopropyl)-3-vinyl imidazolium bromide fiber showed higher extraction efficiency than GO-1-(3-aminopropyl)-3-vinyl imidazolium tetrafluoroborate, PA and CAR-PDMS fibers. Coffee was also analyzed | [52] |
DEP, DPP, DAP, DBP, BBP, and DEHP | Water (- mL plus 20% w/v NaCl) | SPME using a OH-TPB-COFs fiber, stirring at 105°C in HS mode for 50 min, and desorption at 250°C for 7 min | GC-FID | 0.11–1.50 μg/L | 78.6–101.9% at 1 and 5 μg/L | Three sample were analyzed and contained at least 4 PAEs at levels from 1.39 to 5.78 μg/L | OH-TPB-COFs fiber showed better extraction efficiency than PDMS fiber | [46] |
DMP, DBP, DINP, DEP, BBP, DEHP, DNOP, and DIDP | Mineral water (9 mL) | IT-SPME using AC-PS-DVB monolithic columns, and desorption with 1.5 mL ACN | CE-DAD, UHPLC-UV | 0.59–9.83 μg/L | 78.8–104.6% at 50 μg/L | One sample was analyzed, and no residues were detected | AC-PS-DVB monolithic column showed better extraction efficiency than AC-poly(BMA-EDMA) monolithic column. ACN showed higher extraction efficiency than MeOH as desorption solvent. | [62] |
DMP, DEP, DAP, BBP, DBP, DNPP, and DCHP | Disposable tableware, plastic cup and river waters (45 mL plus 2% v/v MeOH) | IT-SPME using PDA-melamineformaldehyde aerogel-carbonfiber tube, and desorption with MeOH-water for 0.6 mL | HPLC-DAD | 0.07–0.16 μg/L | 77–120% at 10 and 15 μg/L | One sample of each water were analyzed and residues of DAP, BBP and DNPP were found at levels from 0.12 to 0.99 μg/L in the water in plastic cup | PDA-melamineformaldehyde aerogelcarbon-fiber tube showed better extraction efficiency than melamine-formaldehyde aerogel-carbon-fiber and bare carbon-fiber tubes | [23] |
SBSE | ||||||||
DMP, DEP, DBP, BBP, DEHP, and DNOP | Sea and esturiane waters (20 mL plus 30% w/v NaCl and 20% v/v MeOH) | SBSE using a PDMS stir bar, stirring at room temperature for 60–200 min, and thermal desorption at 300°C for 10 min | GC-MS | 0.0003–0.063 μg/L | 95–124% at 0.1 μg/L | One river water sample and 2 estuarian water samples were analyzed and contained all PAEs at levels from 0.0036 ± 0.0004 to 1.314 ± 0.018 μg/L | A Plackett–Burman and 2 central composite designs were used for optimization purposes. 6 polycyclic aromatic hydrocarbons, 12 polychlorinated biphenyls and 3 nonylphenols were also analyzed | [65] |
DMP, DEP, DIBP, DBP, DMEP, DMPP, DEEP, DNPP, DHXP, BBP DBEP, DCHP, DEHP, DPhP, and DNOP | Sea water (25 mL plus 5% w/v NaCl and 10% v/v MeOH) | SBSE using a PDMS stir bar, stirring at room temperature for 120 min, and desorption with 200 μL MeOH and 50 μL ACN by sonication for 50 min | GC-MS | 0.00027–1.63 μg/L | - | No samples were analyzed | The stir bar coated with 150 μl PDMS showed higher extraction efficiency than coated with 50 μL and 75 μL PDMS, and 150 μL PDMS over carbon film. A mix MeOH-ACN showed higher extraction efficiency than MeOH and dichloromethane as desorption solvent | [66] |
μ-ECD, micro-electron capture detector; AC, activated carbon; ACN, acetonitrile; BBP, benzylbutyl phthalate; BMA, butyl methacrylate; BMPP, bis(4-methyl-2-pentyl) phthalate; CAR, carboxen; CE, capillary electrophoresis; COFs, covalent organic frameworks; CW, carbowax; DAD, diode-array detector; DAP, diallyl phthalate; DBEP, di(2-butoxyethyl) phthalate; DBP, dibutyl phthalate; DCHP, dicyclohexyl phthalate; DEEP, di(2-ethoxyethyl) phthalate; DEHA, di(2-ethylhexyl) adipate; DEHP, di(2-ethylhexyl) phthalate; DEP, diethyl phthalate; DHXP, dihexyl phthalate; DI, direct immersion; DIBP, diisobutyl phthalate; DIDP, diisodecyl phthalate; DINP, diisononyl phthalate; DIPP, diisopentyl phthalate; DMEP, di(2-methoxyethyl) phthalate; DMP, dimethyl phthalate; DMPP, dimethylethyl phthalate; DNOP, di-n-octyl phthalate; DNPP, di-n-pentyl phthalate; DPhP, diphenyl phthalate; DPP, dipropyl phthalate; DVB, divinylbenzene; EDMA, ethylene dimethacrylate; FID, flame ionization detector; G, graphene; GC, gas chromatography; GO, graphene oxide; HPLC, high-performance liquid chromatography; HS, headspace; IT-SPME, in tube-solid-phase microextraction; LOQ, limit of quantification; MeOH, methanol; MIP, molecularly imprinted polymer; MS/MS, tandem mass spectrometry; MS, mass spectrometry; MWCNTs, multi-walled carbon nanotubes; NPs, nanoparticles; PA, polyacrylate; PAE, phthalic acid ester; PDA, poly(dopamine); PDMS, polydimethylsiloxane; PET, polyethylene terephthalate; PPy, polypyrrole; PS, polystyrene; PVC, polyvinylchloride; SBSE, stir bar sorptive extraction; SPE, solidphase extraction; SPME, solid-phase microextraction; TPB, 2,4,6-triphenoxy-1,3,5-benzene; UHPLC, ultra-performance liquid chromatography; UV, ultraviolet.
Читать дальшеИнтервал:
Закладка:
Похожие книги на «Applied Water Science»
Представляем Вашему вниманию похожие книги на «Applied Water Science» списком для выбора. Мы отобрали схожую по названию и смыслу литературу в надежде предоставить читателям больше вариантов отыскать новые, интересные, ещё непрочитанные произведения.
Обсуждение, отзывы о книге «Applied Water Science» и просто собственные мнения читателей. Оставьте ваши комментарии, напишите, что Вы думаете о произведении, его смысле или главных героях. Укажите что конкретно понравилось, а что нет, и почему Вы так считаете.