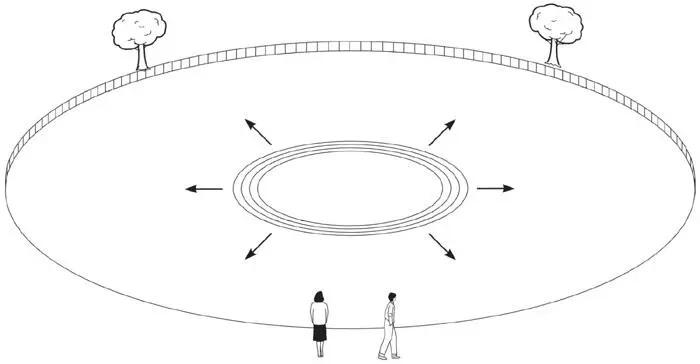
Figure 24.The pond argument. According to the spectator standing on the bank, the ripples move to the left and right with equal speed. But her partner, walking along the bank, sees things differently. He can almost keep up with the waves going to the right, while the left-moving waves recede from him almost twice as fast.
It was soon seen that the aether should introduce an experimentally verifiable standard of rest. The argument is simple and seems irrefutable (Figure 24). If you throw a stone onto the still surface of a pond, waves spread out in concentric rings. The water molecules do not move with the wave, they merely go up and down. The water plays the role of the conjectured aether: itself at rest, it is the material carrier of the waves. As seen by the woman standing on the bank, the waves spread out in all directions with the same speed. But for her partner walking along the bank, the wave process unfolds differently. The waves moving in the same direction as him have a different velocity relative to him compared with the waves moving in the opposite direction. A fast walker will even overtake some of the waves. The relativity principle cannot hold for such processes, and it was therefore expected that it would hold only for the mechanical processes described by Newton’s laws, but not for optical and electromagnetic phenomena. Moreover, as the Earth revolves in its orbit around the Sun it must be continually changing its speed through the aether. This ought to result in observable effects.
In fact, the argument is not quite so simple. Everyone agreed that light must be carried by an aether, but were all parts of the aether at rest relative to one another? As the Earth orbits the Sun, might it not carry some aether with it? It was also necessary to consider what would happen to light passing through water flowing on the Earth. Would the aether be carried along, partially or completely, by the water relative to the Earth? In fact, many issues had to be considered, including aberration, which makes the stars seem to shift slightly towards the point in the sky towards which the Earth is moving at any instant as it orbits the Sun. The arguments, some of which predated Maxwell’s work, developed over a period of eighty years, and many important experiments were made. By 1895, when the Dutch physicist Hendrik Anton Lorentz published an influential study, a consensus had more or less developed. It was that all known experimental results, with one crucial exception, could be explained by assuming the existence of a perfectly rigid aether.
The aether as proposed by Lorentz was actually devoid of all physical properties except rigidity. It was simply there to carry the excitations of Maxwell’s electromagnetic field and, in Lorentz’s words, to be the framework ‘relative to which all observable motions of the celestial bodies take place’. It therefore supplied a standard of rest like the water in the pond.
The one exception with which Lorentz had to contend was the famous Michelson-Morley experiment performed with great accuracy in 1887. Based on interference between light beams moving in the direction of the Earth’s motion and at right angles to it, it was designed to measure the change in the speed of the Earth’s motion through the aether over the course of a year. Its accuracy was sufficient to detect even only one-hundredth of the expected effect. But nothing was observed. It was a great surprise, and very puzzling.
Lorentz’s response was piecemeal. In particular, he suggested that, for some physical reason, the length of a body moving relative to the aether could be reduced in the direction of its motion by the amount needed to explain the Michelson-Morley result. Some years earlier, the Irish physicist George Fitzgerald had made the same proposal. Poincaré responded that some general principle should rule out all possibility of detecting motion relative to the aether. It should not be necessary to invoke ad hoc hypotheses. He began to think that the relativity principle might hold universally and not just for mechanical phenomena. Both he and Lorentz were working in this direction when Einstein appeared on the scene with a stunning solution.
EINSTEIN AND SIMULTANEITY
Two aspects of Einstein’s work ensured its triumphant success. First, he took the relativity principle utterly seriously. It was the bedrock, repeatedly exploited. Second, he took for real a ‘local time’ that Lorentz had introduced as a formal device to describe phenomena in a reference frame moving relative to the aether. Events simultaneous in the ‘local time’ were not so in the real time of the aether frame. But Einstein, committed to relativity, regarded one as just as real as the other. He made a virtue out of an apparent vice, and saw that the key to the entire mystery lay in the concept of simultaneity.
He deliberately highlighted an apparently irreconcilable paradox and then deftly presented its unique resolution: a radical proposal for saying when events are simultaneous. Hitherto this had seemed obvious, but Einstein showed that simultaneity was not a property of the world but a reflection of the way we describe it. By showing that the paradox could be resolved only by changing the notion of simultaneity – and with it time – he brought this issue to the fore.
The paradox was carefully prepared. He first defined the relativity principle. As in mechanics (Box 5), he postulated distinguished frames of reference in which all the laws of nature take their simplest form, and required this form to be the same in each frame. Any such frame, which constituted a kind of grid in space and time, should be in a state of uniform rectilinear (i.e. straight) motion relative to any other. He then postulated, in addition to this general principle, just one actual law of nature: that light propagates with the same speed c in all directions irrespective of the speed of the source. This was exactly what everybody had always assumed would hold in the unique frame of reference at rest in the aether. Einstein insisted it should happen in all frames.
The pond argument suggests that this is absurd. But Einstein realized that he had a hitherto unrecognized freedom: the grid lines defining simultaneity in space and time could be ‘drawn’ in a novel way. Simultaneity at spatially separated points must be defined in some way – but how? There must be a physical transmission of signals so as to synchronize clocks. The ideal would be infinitely fast signals. Then there would be no argument. This is effectively what happens in the pond experiment – the man and woman observe the water waves by light, which travels nearly a billion times faster than they do. We now see that the analogy between water waves in ponds and light waves in the aether is not perfect. For a full analogy, there would have to be signals that travel faster than light itself.
But such signals were unknown in Einstein’s time, and his theory would show that they could not exist. He therefore used the best substitute – light. This completely changed things. Light was to be analysed in a framework that light itself created, so the problem became self-referential. It might seem that Einstein cheated, making up the rules as he went along to ensure that he won the game. However, he was simply confronting a fact of life: laws of nature will be meaningful only if they relate things that can actually be observed. We live inside, not outside, the universe, and to synchronize distant clocks we have no alternative to the physical means available to us. Einstein’s hunch that we should use light because it would turn out to be the fastest medium available in nature has so far been totally vindicated.
Читать дальше