Subsurface water can be split into two distinct zones: saturation zone and aeration zone .
This is the depth throughout which all the fissures and voids are filled with water under pressure. The upper level of this water is known as the groundwater table (GWT) , phreatic surface or groundwater level (GWL) , and the water within this zone is called phreatic water or, more commonly, groundwater .
The water table tends to follow, in a more gentle manner, the topographical features of the surface above ( Fig. 2.1). At groundwater level, the hydrostatic pressure is zero, so another definition of water table is the level to which water will eventually rise in an unlined borehole.
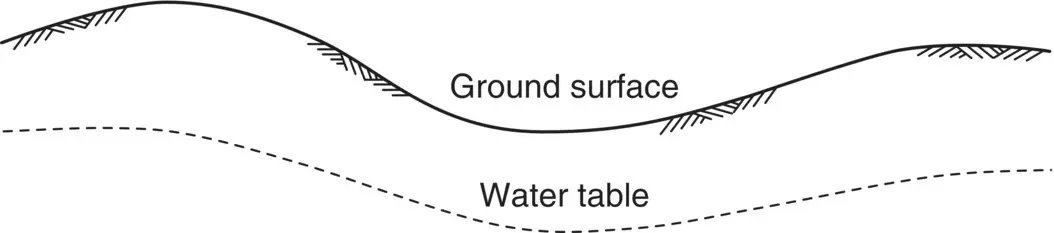
Fig. 2.1 Tendency of the water table to follow the earth's surface.
The water table is not constant in depth but rises and falls with variations of rainfall, atmospheric pressure, temperature, and proximity to tree roots. In coastal regions, the GWL can be affected by tides and is said to be tidal. At locations where the water table reaches the ground surface, springs, lakes, swamps, and similar features can be formed.
Sometimes referred to as the vadose zone , this zone occurs between the water table and the surface, and can be split into three sections.
Owing to capillarity, water is drawn up above the water table into the voids of the soil. Water in this fringe can be regarded as being in a state of negative pressure, i.e. at pressure values below atmospheric. The minimum height of the fringe is governed by the maximum size of the voids within the soil. Up to this height above the water table, the soil will be sufficiently close to full saturation to be considered as such. The maximum height of the fringe is governed by the minimum size of the voids. Between the minimum and maximum heights, the soil is partially saturated .
Terzaghi and Peck (1948) give an approximate relationship between the maximum height and the grain size for a granular soil:
where C is a constant depending upon the shape of the grains and the surface impurities (varying from 10.0 to 50.0 mm 2), and D 10is the effective size expressed in millimetres.
As rainwater percolates downward to the water table, a certain amount is held in the soil by the action of surface tension, capillarity, adsorption, and chemical action. The water retained in this manner is termed held water and is deep enough not to be affected by plants.
This zone is constantly affected by precipitation, evaporation, and plant transpiration. Moist soil in contact with the atmosphere either evaporates water or condenses water into itself until its vapour pressure is equal to atmospheric pressure. Soil water in atmospheric equilibrium is called hygroscopic water and its water content (which depends upon relative humidity) is known as the hygroscopic water content .
The various zones are illustrated in Fig. 2.2
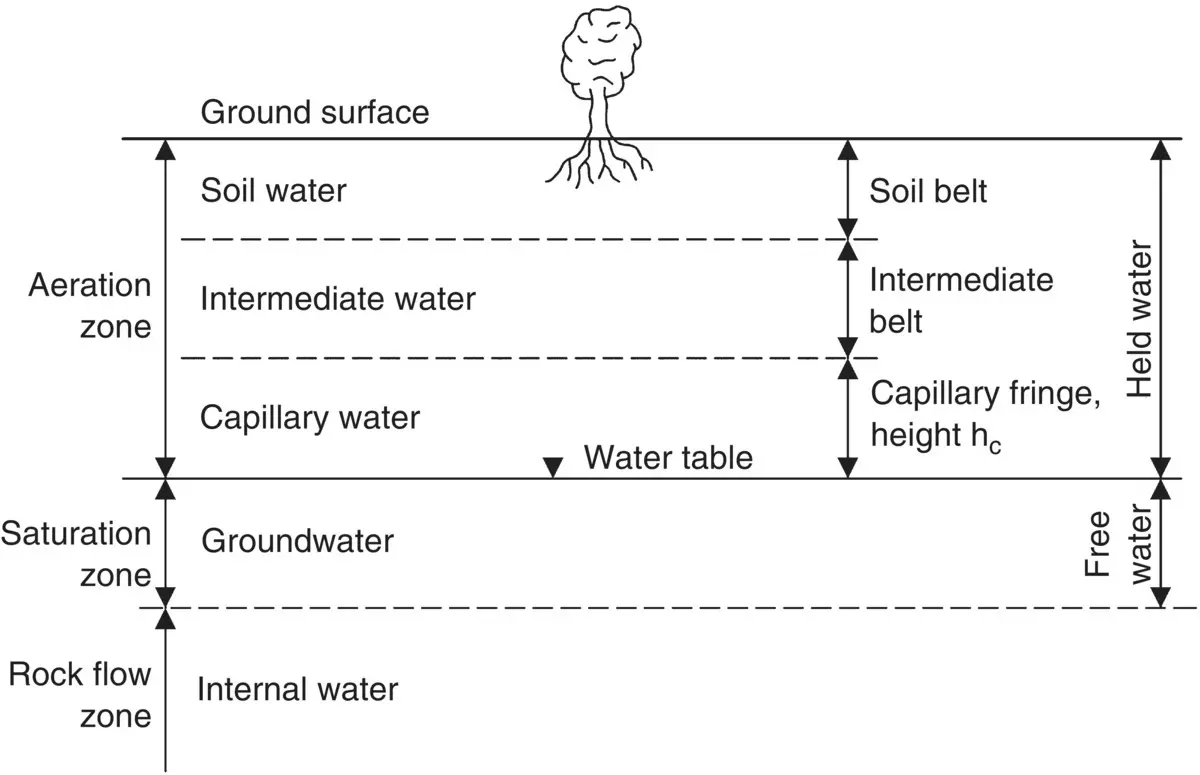
Fig. 2.2 Types of subsurface water.
2.2 Flow of water through soils
The voids of a soil (and of most rocks) are connected together and form continuous passageways for the movement of water brought about by rainfall infiltration, transpiration of plants, imbalance of chemical energy, or a variation of the intensity of dissolved salts.
When rainfall falls on the soil surface, some of the water infiltrates the surface and percolates downward through the soil. This downward flow results from a gravitational force acting on the water. During flow, some of the water is held in the voids in the aeration zone and the remainder reaches the groundwater table and the saturation zone. In the aeration zone, flow is said to be unsaturated . Below the water table, flow is said to be saturated . Flow of water through soils is often referred to as seepage .
The water within the voids of a soil is under pressure. This water, known as pore water , may be static or flowing. Water in saturated soil will flow in response to variations in hydraulic head within the soil mass. These variations may be natural or induced by excavation or construction.
The head of water acting at a point in a submerged soil mass is known as the hydraulic head and is expressed by Bernoulli's equation:
(2.1) 
In seepage problems, atmospheric pressure is taken as zero and the velocity is so small that the velocity head becomes negligible; the hydraulic head is therefore taken as:
(2.2) 
Water flows from points of high to points of low head. Hence, flow will occur between two points if the hydraulic head at one is less than the head at the other, and in flowing between the points, the water experiences a head loss equal to the difference in head between them. This difference is known as the excess head .
The conduits of a soil are irregular and of small diameter – an average value of the diameter is D 10/5. Any flow quantities calculated by the theory of pipe flow must be in error and it is necessary to think in terms of an average velocity through a given area of soil rather than specific velocities through particular conduits.
If Q is the quantity of flow passing through an area A in time t, then the average velocity, v is:
(2.3) 
This average velocity is sometimes referred to as the seepage velocity . In further work, the term velocity will imply average velocity.
If we install two vertical standpipes, a distance apart and with their lower ends embedded into a zone of saturated soil, groundwater will rise in the pipes to different levels as shown in Fig. 2.3. (A standpipe is simply an open‐ended tube, perforated at its lower end, which permits groundwater to flow into the tube and to rise to a final position. See Section 7.5.1 for more details.)
Akin to the equation of a straight line in mathematics, the hydraulic gradient is defined as the difference in vertical values divided by the difference in horizontal values, at the two points considered.
i.e.
(2.4) 
Читать дальше