This may seem bass-ackwards, I admit. Why not just build an artificial notochord and then add plastic rings, as the game of life demands, to model the number of vertebrae? Our rationale for evolving bending stiffness as a proxy for vertebrae went as follows. If you evolve only whole vertebrae—they are either present or absent—then your resolution is limited to those stepwise changes. You can’t see what “half” a vertebrae looks like. But do half-vertebrae evolve? Yes, sometimes. In the fossil record for the group of fleshy-finned fishes that were the outgroups to the first land-living tetrapods, we see partial ring vertebrae, little crescents of bone that cup the bottoms of the notochord. [24] For reconstructions of the skeletons and their partial vertebrae, see J. A. Long and M. S. Gordon, “The Greatest Step in Vertebrate History: A Paleobiological Review of the Fish-Tetrapod Transition,” Physiological and Biochemical Zoology 77, no. 5 (2004): 700–719.
At least in this group, it looks like vertebrae form from different pre-existing centers of bone formation, in this case the ribs. Sindre Grotmol and his colleagues at the University of Bergen, Norway, have shown a similar process in the development of living fishes.
Here’s the rub if, like us, you are interested in evolutionary biorobotics: how do you make partial vertebrae? We’ve tried, trust me. My students can tell you many a tale of working on making tails with partial vertebrae and vertebrae of various sizes and shapes. But in almost every case the vertebral column would tear (fracture, strictly speaking) at the interface between the bit of vertebra and the notochord.
Our solution, at the time, was to forget about the vertebrae and make a continuous structure, a biomimetic notochord whose material stiffness we could alter and, in so doing, alter the notochord’s flexural stiffness. [25] The use of the term “biomimetic” varies across engineering, bioengineering, and biomedical engineering. Here I use “biomimetic” to mean a system built to resemble, as much as possible, the biological target.
We also realized that if you changed the length of a structure, you alter its structural stiffness: for a given flexural stiffness, a longer structure deflects more than a shorter one. Our biomimetic notochord was, in the lingo of material scientists, a hydrogel made, as I said, of collagen, thanks to Tom Koob.
When you take the powdered gelatin and add it to heated water, it dissolves nicely if you stir the pot. As you cool the mixture, the gelatin, now evenly spread throughout the forming solid, makes some chemical bonds between the scattered molecules. Pour the cooling liquid into a mold of some kind and pop it into the refrigerator. In the cold the motion of the collagen fragments slows, allowing even more bonds to form. Presto! You’ve created a solid from a liquid: a molded hydrogel!
For biomimetic hydrogels, we poured the hot gelatin and water mixture into an array of molds that made cylindrical rods about 10 centimeters in length and about 0.5 centimeters in diameter. Once the gelatin had set in the fridge, we pulled the rods out and then did something you wouldn’t do with your dessert: chemically embalm them. Embalming, or what a biochemist would call fixation or, in this case, cross-linking, keeps tissues from degrading and, gulp, spoiling.
For our hydrogels, the mortuarial embalming agent we use is called glutaraldehyde, and it does two things. First, glutaraldehyde allows us to let the biomimetic notochords warm up to room temperature without melting—it keeps the hydrogels solid. Second, glutaraldehyde allows us to control the stiffness of the hydrogel: the more time that the hydrogel spends in the glutaraldehyde solution, the stiffer it becomes as more chemical crosslinks form between collagen molecules. Here, finally, was our method for getting any intermediate flexural stiffness that a genetic call for a partial vertebra might require.
DESIGN QUESTION 3: WHICH FEATURES OF THE ANIMAL’S WORLD WILL WE MODEL AND WHY?
The world or arena that you design for your robots is as important as the robots themselves. Thus, we have in hand one of the design principles for embodied robots expounded by Rohlf Pfeifer and Cristian Scheier: build a robot for a specific ecological niche. [26] Rolf Pfeifer, and Christian Scheier, Understanding Intelligence (Cambridge, MA: MIT Press, 1999).
In other words, you have to build the agent with a particular world in mind. This is obvious when we think about the difference between a fish-like robot and a dog-like robot: water versus land. But what about a fish-like robot swimming in the nooks and crannies of a coral reef and one swimming in the open ocean? If we use fish as our guides, these robots ought to be very different kinds of agents, the first skilled at precise maneuvering and station-holding and the second skilled at cruising and perhaps navigation.
The world also has other players. For evolutionary biologists, the other players are called “biotic factors” and everything else is “abiotic factors.” For an individual robot, biotic factors are all the other robots and animals with which it might interact. Abiotic factors include the physical and chemical situation in which it’s placed. Together, biotic and abiotic factors make up the ecological niche, here what I’m calling the stage, the modeled world, or the selection environment.
We wanted a world that, like Tadro itself, was a simplification of our best-guess of ancient reality. The ancient world for the first vertebrates was, as far as we can tell, oceanic, near the shore, and full of biotic factors like giant arthropods, trilobites, anemones, and worms with legs. [27] Hou Xian-Guang, Richard J. Aldridge, Jan Bergstron, David J. Siveter, Derek J. Siveter, and Feng Xiang-Hong, The Cambrian Fossils of Chengjiang, China: The Flowering of Early Animal Life (Malden, MA: Wiley-Blackwell, 2007).
Obviously they all had to eat, and some of them likely competed with the first vertebrates, jostling for position at the donut store, figuratively speaking. KISS demanded we leave most of that cast of characters out.
The simple world we built was a water world, a walled tank 2.5 meters across with a single sun, limited time, and three Tadro3s (Figure 3.4). The sun was a hundred-watt flood light suspended above the surface of the water. Time was limited to three minutes for each trial. Each Tadro3 competed in six different trials, with three robots in each trial. To account for the fact that each Tadro3, even though built to be identical in every way but for their variable tails, may vary in performance, we swapped the biomimetic tails among the three Tadro3s and made sure that all possible combinations of tails and robots were tested. This swapping allowed us to make sure that no particular tail lost the game because it was always stuck with a sluggish robot.
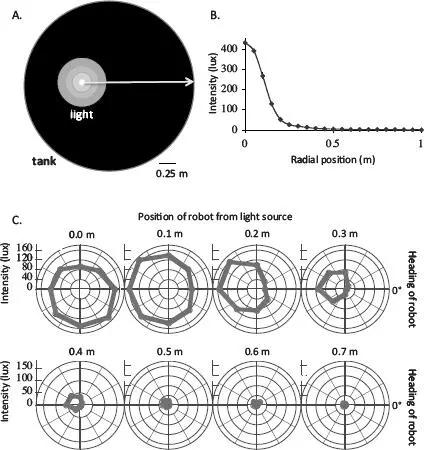
FIGURE 3.4. The water world of Tadro3. A. Overhead view of the surface of the water in the 2.5 meter-diameter circular tank, with the position and distribution of the light and its gradient shown by the nested circles. The big white arrow indicates a radial slice of the light gradient shown in B. C. Perception of the light gradient by Tadro3s. Polar plots indicate light intensity (along radii, with origin at 0 lux) registered by robots at different headings every 0.1 meters along the radial slice shown in B. A heading of 0 degrees means that the robot was facing in the direction indicated by the arrow in A. Recall that eyespot is located 45 degrees to the left of the robot’s center line (see Figure 3.3).
Читать дальше