3.4.2 Ambient Assisted Living and Building Automatization
The concept of ambient assisted living is strongly related to building automation, smart homes, and the IoT. The idea is to support humans in their homes with automated and often customized electronic assistance, often with a focus on the elderly or handicapped individuals. In most applications, reliability is critical. That said, with ambient assisted living still in its early stage of development, this application with its many required sensors has huge potential for IPV; however, so far, the market coverage is very low.
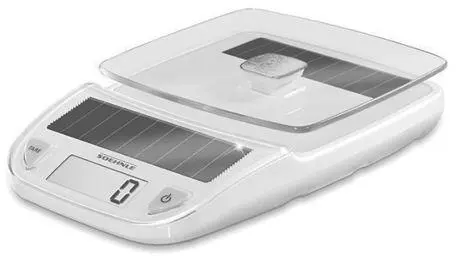
Figure 3.3Solar powered kitchen scale. (Photo reproduced by permission of Soehnle-Leifheit, 2019).
The aim of building automation is to provide comfort and efficiency and to automate energy-saving measures, such as switching lights on and off or regulating room temperatures. Besides financial losses or discomfort, a malfunction of a sensor poses no significant risks. On the other hand, installing automated energy-saving technologies will pay off for most applications very quickly. EnOcean provides different batteryless IPV powered sensors in this application field [33].
3.4.3 Industry, Agriculture, Horticulture, Retail, and Logistics
These applications show some of the highest potential for a significant market growth of IPV, as they are classical applications for IoT and cloud-assisted AI solutions. Typically, the IoT edge node needs to transmit or receive a sensor or actor signal in a regular period or even only under certain circumstances. Therefore, the required average power consumption of such an IoT edge node can range below a few milli- or even micro-watts, which can be provided from photovoltaic generators in most cases. There are different optimized products available on the market, including electronics in the case of the TI Bluetooth Low Energy Beacon [34]. IXYS specializes in manufacturing high-efficiency crystalline silicon modules that have been proven to be reliable indoors [10, 35]. The challenge and in some cases the advantage for some of these applications is the frequency of incident radiation. Applications with a typical schedule, such as production hours or even automated and spectrally defined radiation, such as in horticultural application, are an ideal case for IPV design due to their known operating conditions.
Logistics and retail have often been discussed as possible fields of application for micro energy harvesting. However, these applications often suffer from sufficient radiation and/or recycling issues in single-use applications, such as in tracking logistics. As proposed in the introduction, a dedicated radiation power beam might be an approach to some of those applications.
3.4.4 Relation of IPV to Outdoor Applications – Hiking, Emergency Kits
Many small-scale products available on the market were designed for outdoor use or outdoor charging. Among these are emergency kits for hikers, and phone chargers, torches and lamps such as the Little Sun products or the SOLVINDEN table lamp by IKEA [36, 37]. Generally, the module size of these products is a few square centimeters and the material is crystalline or amorphous Si. Although some products use high efficiency cells, they are not optimized for the spectral indoor conditions in their distribution and intensity. Other outdoor-related applications include solar fans for camping or solar power LED lamps. The module sizes for these applications already come close to the range of square meters.
3.5 Degradation and Lifetime Issues
Degradation effects decrease the efficiency over time, thus limit the lifetime among other effects. As most degradation effects are related to energy and intensity levels, indoor conditions are again in favor of the photovoltaic materials. The Stabler-Wronski-Effect in a:Si:H is related to intensity [38], so a:Si:H can expect a longer lifetime indoors than in outdoor operation. As the first generations of outdoor systems start to report lifetimes above three decades, for most materials the photovoltaic module will not be the limiting device for the total device lifetime in IoT edge nodes.
However, so far, few studies have been reported investigating the lifetime for IPV products. In a study from 2013, photodiodes from GaAsP and organic solar cells (P3HT:PCBM) were irradiated by fluorescent light for 3900 hours [39]. This would be approximately 2.3 years of use in an office with 222 working days per year and 8 hours of artificial irradiance per day. The organic cells degraded about 15% from their initial efficiency, the GaAsP about 10%. As these devices might be installed for a long time, degradation issues need to be addressed during design.
A second topic in product design is recycling and toxicity. As IoT devices are small, they might be forgotten in their installations or be integrated into building structure and furniture. Easy removal and recycling and avoidance or handling of toxicity is thus important during early design. Finally, the total cost of ownership and the CO 2footprint of those devices need to be addressed over the whole production chain and life cycle. Reinders and Apostolou provided an overview of product integrated PV, including IPV and outdoor applications, such as in cars [40]. Chapter 8introduces current IPV product integration.
3.6 Conclusions and Outlook
The recent takeoff of the IoT could enable the takeoff of IPV. This chapter showed its large potential, and issues to be solved on the way to a safe and secure mass production enabling the full efficiency and reliability of IPV-powered products. The time when we might start to wonder why devices were not powered by IPV might not be so far away.
1 1. Hyatt, G.P., Single chip integrated circuit computer architecture. US Patent 4942516A, 28, Dec. 1970.
2 2. Faggin, F., Hoff, M., Mazor, S., Memory system for a multi chip digital computer, vol. 22, Intel Corp, US Patent 3821715A, Jan. 1973.
3 3. Conti, J.A., Electronic postage weighing scale, vol. 10, Pitney-Bowes Inc, US Patent 4084242A, Nov. 1976.
4 4. Roen, S.A., Solar powered portable calculator, vol. 03, Litton Business Systems Inc, US Patent 4017725A, Jan. 1975.
5 5. Hanson, S. et al., IEEE J. Solid-State Circuits., 44, 1145–1155, 2009.
6 6. Atluri, V. et al., The trillion-dollar opportunity for the industrial sector. McKinsey Digital, November 2018. Article https://www.mckinsey.com/business-functions/mckinsey-digital/our-insights/the-trillion-dollar-opportunity-for-the-industrial-sector, accessed 2020-01-24.
7 7. IEC 60904-3:2019, Photovoltaic devices - Part 3: Measurement principles for terrestrial photovoltaic (PV) solar devices with reference spectral irradiance data, 2019.
8 8. Randall, J.F. and Jacot, J., Is AM1.5 applicable in practice? Modelling eight photovoltaic materials with respect to light intensity and two spectra. Ren. Energy, 28, 12, 1851–1864, 2003.
9 9. Hirata, Y. et al., Variation of output with environmental factors, in: Photovoltaic Modeling Handbook, M. Freunek Müller (Ed.), Wiley Scrivener, Hoboken NJ, USA, 2018.
10 10. Freunek, M. et al., Maximum efficiencies of indoor photovoltaic devices. IEE J. Photovol., 3, 1, 59–64, 2013.
11 11. Roth, W., Photovoltaische Energieversorgung für Geräte im kleinen und mittleren Leistungsbereich, report, Fraunhofer-Institut für Solare Energiesysteme, Freiburg, Germany, 1991.
12 12. Müller, M. et al., Characterization of indoor photovoltaic devices and light, in: Proceedings of the 34th IEEE Photovoltaic Specialists Conference, pp. 738– 743, Philadelphia, USA, 2009.
Читать дальше