Solar-to-Chemical Conversion
Здесь есть возможность читать онлайн «Solar-to-Chemical Conversion» — ознакомительный отрывок электронной книги совершенно бесплатно, а после прочтения отрывка купить полную версию. В некоторых случаях можно слушать аудио, скачать через торрент в формате fb2 и присутствует краткое содержание. Жанр: unrecognised, на английском языке. Описание произведения, (предисловие) а так же отзывы посетителей доступны на портале библиотеки ЛибКат.
- Название:Solar-to-Chemical Conversion
- Автор:
- Жанр:
- Год:неизвестен
- ISBN:нет данных
- Рейтинг книги:3 / 5. Голосов: 1
-
Избранное:Добавить в избранное
- Отзывы:
-
Ваша оценка:
- 60
- 1
- 2
- 3
- 4
- 5
Solar-to-Chemical Conversion: краткое содержание, описание и аннотация
Предлагаем к чтению аннотацию, описание, краткое содержание или предисловие (зависит от того, что написал сам автор книги «Solar-to-Chemical Conversion»). Если вы не нашли необходимую информацию о книге — напишите в комментариях, мы постараемся отыскать её.
Solar-to-Chemical Conversion — читать онлайн ознакомительный отрывок
Ниже представлен текст книги, разбитый по страницам. Система сохранения места последней прочитанной страницы, позволяет с удобством читать онлайн бесплатно книгу «Solar-to-Chemical Conversion», без необходимости каждый раз заново искать на чём Вы остановились. Поставьте закладку, и сможете в любой момент перейти на страницу, на которой закончили чтение.
Интервал:
Закладка:
In addition, Pastrana‐Martínez et al. prepared a graphene derivative–TiO 2composites for photocatalytic water reduction of CO 2into renewable fuels under UV–vis light irradiation [62]. Meanwhile, the findings present that the pH of solution has significant influence toward selective ethanol formation. The prepared GO/TiO 2composite exhibited superior photocatalytic activity for EtOH production (144.7 μmol g cat −1h −1) at pH 11.0 and for MeOH production (47.0 μmol g cat −1h −1) at pH 4.0. It is found that the yield of C2 hydrocarbons is much lower than that of C1 products due to the demand of more electrons and complicated reaction mechanism, which cannot be varied. Therefore, it is more feasible that the long‐chain hydrocarbons are formed as secondary products over photocatalysts that can realize efficient C1–C2 conversion in the future.
2.4.1.6 Other Hydrocarbons
Apart from these hydrocarbons produced in CO 2photoreduction with high frequency, some complicated hydrocarbons with more than two carbon numbers have been reported recently. Fusco et al. reported firstly the formation of C2 hydrocarbons, acetic acid, through CO 2photoreduction over TiO 2@PEI‐grafted‐MWCNTs hybrids under UV–Vis light irradiation [63]. Besides, Park et al. decorated Cu nanoparticles and CdS quantum dots on the surface of TiO 2nanotubes, forming a ternary nanostructured photocatalyst that is capable of converting CO 2and H 2O into C1–C3 hydrocarbons, including CH 4, C 2H 6, C 3H 6, and C 3H 8( Figure 2.12b–e) [64]. In this system, CdS quantum dots are responsible of harvesting solar light and forming hot electrons that will rapidly move to Cu nanoparticles through TiO 2nanotubes. At Cu nanoparticles, the concentrated electrons can react with CO 2molecular and produce C xH yunder visible‐light excitation (above 420 nm), as shown in Figure 2.12a. In contrast, over five hours irradiation, free H 2molecular that is more easily formed than CO 2reduction products was not detected, which inferred that competitive H 2evolution reaction has been suppressed efficiently in this CdS/(Cu–TNTs) hybrid system. The photocatalytic reduction of CO 2over the CdS/(Cu–TNTs) hybrid is initiated likely through a one‐electron reduction to form ˙CO 2 −, reacting in turn with a H atom (H·) to produce hydrocarbons ( Figure 2.12f). CO 2hydrogenation leading to hydrocarbon formation appears to be a less likely pathway because of the absence of H 2detected in the headspace of the photolysis reactor during five hours of irradiation with visible light. However, the yield and efficiency are sharply decreased owing to involvement of more electrons, formation of longer C–C chains, and production of more by‐products, which severely undermine the proportion of expected products, such as C3 or C4 hydrocarbons. Therefore, to increase the formation possibility and efficiency of major hydrocarbons, electrocatalytic CO 2reduction is paid attention through introducing more strong hot electrons to react with adsorbed CO 2molecular over a variety of electrocatalysts, leading to longer carbon chain hydrocarbons [65].
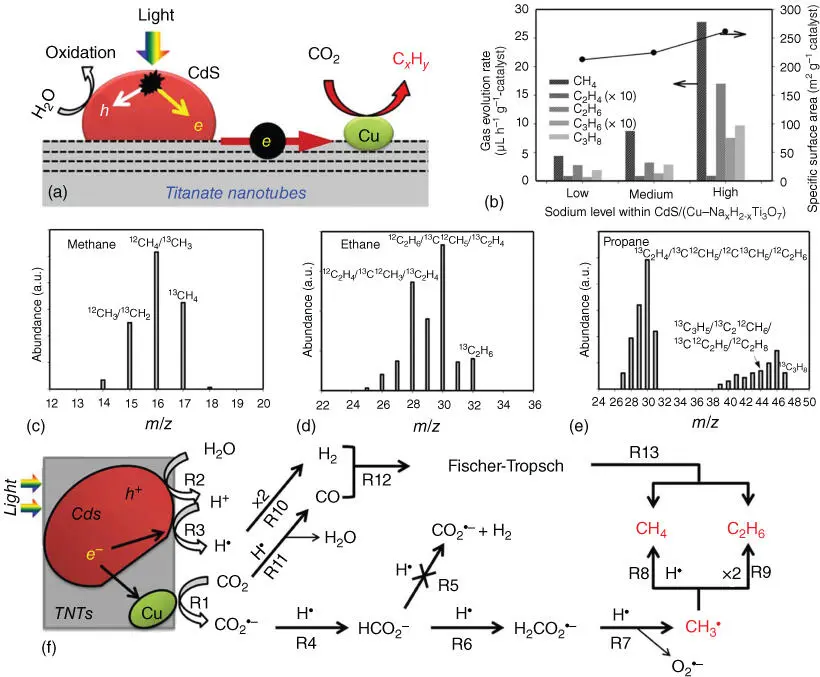
Figure 2.12(a) Scheme of the photocatalytic CO 2reduction over CdS/(Cu–Na xH 2−xTi 3O 7) irradiated by light. (b) Gas evolution rates of C1–C3 hydrocarbons on CdS/(Cu–Na xH 2−xTi 3O 7) as well as the specific surface area, where the Na/Ti ratios were 0.093 (low), 0.143 (medium), and 0.507 (high). (c–e) Mass spectra of the formed hydrocarbons (methane, ethane, and propane) with the labeling of 13C. (f) Proposed elementary reaction mechanisms of photocatalytic CO 2conversion into hydrocarbons.
Source: Park et al. [64].
2.4.2 Carbon Monoxide (CO)
Apart from the hydrocarbons, other carbon‐containing fuels such as CO also are produced as main product in CO 2‐involving photocatalysis [66]. From the view of redox potential, CO formation reaction (−0.48 eV vs. NHE) is inferior to the production of CH 4(−0.38 eV vs. NHE) and CH 3OH (−0.24 eV vs. NHE), while the formation reactions of CH 4and CH 3OH involve six‐ and eight‐electron reaction process, in which a series of elemental reaction are unclear and slow. In contrast, carbon monoxide is simpler than CH 4, CH 3OH, and other hydrocarbons, which means fewer needs of reductive electrons. Therefore, the CO reduction reaction is thermodynamically triggered, and CO is a preferred product in CO 2photoreduction. For instance, Miyauchi and coworkers reported CuO‐decorated Nb 3O 8nanosheets for photocatalytic CO 2reduced into CO, and simultaneously the reaction pathway over this system had been deeply investigated through electron spin resonance (ESR) and isotope‐labeled experiments [67]. The results indicate that amorphous copper oxide nanoclusters can work as efficient electrocatalysts grafted onto the surface of niobate nanosheets for the reduction of carbon dioxide to carbon monoxide. Furthermore, the photocatalytic activity and reaction pathway of Cu(II)‐grafted Nb 3O 8nanosheets were investigated using ESR analysis and isotope‐labeled molecules (H 2 18O and 13CO 2). The results of the labeling experiments demonstrated that under UV irradiation, electrons are extracted from water to produce oxygen ( 18O 2) and then reduce CO 2to produce 13CO. ESR analysis confirmed that excited holes in the VB of Nb 3O 8nanosheets react with water and that excited electrons in the CB of Nb 3O 8nanosheets are injected into the Cu(II) nanoclusters through the interface and are involved in the reduction of CO 2into CO. The Cu(II) nanocluster‐grafted Nb 3O 8nanosheets are composed of nontoxic and abundant elements and can be facilely synthesized by a wet chemical method. The nanocluster grafting technique described here can be applied for the surface activation of various semiconductor light harvesters, such as metal oxide and/or metal chalcogenides, and is expected to aid in the development of efficient CO 2photoreduction systems. Furthermore, Tahir et al. synthesized Ag nanoparticles/TiO 2nanowires core–shell heterojunction for efficient photoreduction of CO 2to CO in the presence of hydrogen [68]. Ag‐NPs coated over TiO 2NWs exhibited strong absorption of visible light due to localized surface plasmon resonance (LSPR) excitation, trapped electrons, and hindered charge recombination rate. The synergistic effect of Ag‐NPs coated over TiO 2NWs for CO 2conversion was evaluated in a gas‐phase system under UV and visible‐light irradiation. The plasmonic Ag‐NPs/TiO 2NWs demonstrated excellent photoactivity in the reduction of CO 2into CO, CH 4, and CH 3OH under visible‐light irradiation. The results show that 3 wt% Ag‐NPs‐loaded TiO 2NWs was found to be the most active, giving the highest CO evolution of 983 mmol g −1h −1at selectivity 98%. This amount of CO produced was 23 times more than the TiO 2NWs and 109 times larger than the yield of CO produced over the pure TiO 2. More importantly, the quantum yield was substantially enhanced for CO evolution. The LSPR excitation and synergic effect of Ag‐NPs that can effectively accelerate the charge separation were proposed to be responsible for the enhancement of photocatalytic activity. The photostability of Ag‐NPs/TiO 2NWs evidenced in cyclic runs for selective CO production under visible light, yet photoactivity declined over the irradiation time under UV light.
Recently, it is well demonstrated that layered nanomaterials with excellent conductivity, e.g. graphene and C 3N 4, can facilitate to accelerate the charge transportation, which is a promising component to efficiently improve the separation efficiency of photogenerated electrons and holes in traditional semiconducting photocatalysts [69]. For instance, Ye and coworkers constructed a smooth carrier channel in the basal plane of organic polymeric C 3N 4photocatalyst by enriching defects with short and strong C–N chains through a glycine linker so that the photoinduced carrier transfer is apparently enhanced. Based on this, the photoreduction ability of CO is increased by 29.2 times under a simulated sunlight irradiation, as shown in Figure 2.13[70]. Compared with complicated hydrocarbons, H 2has strong competition ability to CO due to the lower potential. Therefore, H 2often consumes more photoexcited electrons and suppresses the CO production. Although H 2is cleaner energy storage form, carbon element is not contained in the energy cycle, which is undesirable to control CO 2level in atmosphere. In other words, as a competitive reaction, two‐electron H 2evolution reaction from water splitting should be suppressed deliberately. In 2011, Kudo and coworkers reported a series of Ag‐modified ALa 4Ti 4O 15(A = Ca, Sr, and Ba) photocatalysts that have 3.79–3.85 eV of band gaps and layered perovskite structures, displaying photocatalytic activities of CO 2reduction to produce CO and HCOOH without any sacrificial reagents [71]. It is reported that d 0‐type metal oxide photocatalysts can catalyze water splitting into H 2and O 2because of high CB positions and wide band gaps, which are expected to be active for CO 2photoreduction at a suitable reaction sites on the surface of photocatalysts. Among these photocatalysts, Ag‐loaded BaLa 4Ti 4O 15showed the best photocatalytic activity. The Ag as a cocatalyst prepared by the liquid‐phase chemical reduction method was loaded as fine particles with the size smaller than 10 nm on the edge of the BaLa 4Ti 4O 15. On the optimized Ag/BaLa 4Ti 4O 15photocatalyst, CO was the main reduction product rather than H 2even in an aqueous medium. Furthermore, evolution of O 2in a stoichiometric ratio (H 2+ CO:O 2= 2 : 1 in a molar ratio) indicated that water was consumed as a reducing reagent (an electron donor) for the CO 2reduction, which demonstrated that CO 2reduction accompanied with water oxidation was achieved using the Ag/BaLa 4Ti 4O 15photocatalyst. After that, Teramura and coworkers designed a new three‐component heterojunction for efficiently hampering the H 2evolution and increasing the selectivity of CO. [72] The results indicated that Zn‐doped Ga 2O 3exhibited significant restraint on H 2releasing from overall water splitting. Based on this result, they further deposited Ag onto the Zn–Ga 2O 3to increase the production efficiency of CO because introduction of Ag as a cocatalyst could enhance the harvesting efficiency of sunlight and collect more photogenerated electrons for CO 2reduction. In the case of Ag/Zn/Ga 2O 3, the selectivity toward CO evolution is higher than toward H 2in the presence of NaHCO 3solution, where the yields of CO and H 2reached to 800 and 60 μmol g cat −1, respectively, over seven hours under UV irradiation. At the same time, the O 2evolution was observed, which inferred that overall water splitting happened in this system while H 2releasing was suppressed. These results indicate that the Ag‐modified Zn‐doped Ga 2O 3realizes selective conversion of CO 2and H 2O to CO and O 2under UV irradiation.
Читать дальшеИнтервал:
Закладка:
Похожие книги на «Solar-to-Chemical Conversion»
Представляем Вашему вниманию похожие книги на «Solar-to-Chemical Conversion» списком для выбора. Мы отобрали схожую по названию и смыслу литературу в надежде предоставить читателям больше вариантов отыскать новые, интересные, ещё непрочитанные произведения.
Обсуждение, отзывы о книге «Solar-to-Chemical Conversion» и просто собственные мнения читателей. Оставьте ваши комментарии, напишите, что Вы думаете о произведении, его смысле или главных героях. Укажите что конкретно понравилось, а что нет, и почему Вы так считаете.