YSZ, yttria‐stabilized zirconia (3 mol% Y 2O 3); ZTA, alumina matrix composite containing 15−20 vol% tetragonal ZrO 2particles; UHMWPE, Ultrahigh molecular weight polyethylene; PMMA, polymethyl methacrylate.
aCP, commercial purity.
bASTM designation.
cStrength increases but elongation to failure decreases from Grade 1 to Grade 4.
//, parallel to long direction.
⊥, perpendicular to long direction.
The negative sign is included because most materials contract in the direction perpendicular to a tensile stress, that is, the strain in the lateral direction is opposite in sign to the strain in the tensile direction. Consequently, ν is positive for most materials. Values for the Poisson’s ratio for the majority of materials are in the range ~0.25–0.40.
There are other forms of elastic behavior, although the stress versus strain curve is not a straight line or the unloading part of the curve does not follow the same path as the loading part. Polymers show elastic behavior in that they recover completely when the stress is removed but the recovery is slow and the stress–strain curve can be linear or nonlinear. Materials such as synthetic and natural rubbers, and the natural material elastin undergo large deformations and when unloaded, they return to their original shape. In spite of this, their stress–strain curve is nonlinear.
4.2.3 Mechanical Response of Materials
Materials show a variety of mechanical response that is strongly dependent on their interatomic bonding and structure. Following perfectly elastic deformation, ceramics (including glasses and glass‐ceramics) typically fracture catastrophically in a brittle manner whereas metals (and their alloys) deform plastically prior to eventual failure. Unlike metals and ceramics, the mechanical response of polymers at a given temperature is strongly dependent on the time over which the stress is applied (or the rate at which the stress is increased). This time‐dependent mechanical response of polymers is called viscoelasticity. Although large changes in temperature are not relevant to the application of biomaterials, it should be noted that unlike metals and ceramics, the mechanical response of polymers is also highly sensitive to the temperature of testing.
Elastic and Plastic Deformation
The measured stress–strain curves of solids show a variety of shapes but the schematic curve in Figure 4.2can be used to illustrate the distinction between elastic and plastic deformation. For metals, perfectly elastic behavior (less than approximately 0.1% strain) is followed by plastic deformation that can continue to much larger strains until the specimen fails, typically by breaking into two pieces. The strain at which the specimen fails, called the elongation to failure, can be in the range ~1% to over 50% for many metals. In comparison, ceramics fail within their elastic region, at strains less than ~0.1%, and the material fractures catastrophically into two or more pieces, a failure mode called brittle fracture. The ability to deform plastically is commonly referred to as ductility and, thus, metals are said to be ductile whereas ceramics are brittle.
Figure 4.2 Schematic stress–strain curve to illustrate the distinction between elastic and plastic deformation of materials.
A key difference between elastic deformation and plastic deformation is that unlike elastic deformation, the strain or elongation due to plastic deformation is not recovered upon unloading the material. Upon loading a specimen from its unloaded dimension (point O in Figure 4.2) to point A in the elastic region, the specimen returns to its original dimension along the same line AO. Upon loading, atoms in the solid are displaced slightly from their equilibrium sites but do not take up new sites ( Chapter 2). When the load is removed, the atoms return to their original equilibrium sites. In comparison, plastic deformation is irreversible. Upon loading a specimen to point B, the specimen follows the line BO′ upon unloading which is approximately parallel to AO. The small elastic portion of the strain is recovered but not the plastic portion. The atoms take up new sites relative to each other by movement of dislocations ( Chapter 3). To summarize at this stage, the key features of elastic and plastic deformation are:
Elastic deformationStrains commonly smallReversible: the material returns to its original unloaded dimensionAtoms are displaced slightly upon deformation but do not take up new sites
Plastic deformationStains much larger than elastic deformationIrreversible: the plastic strain is not recovered upon unloadingAtoms take up new sites relative to each other
Elastic Limit and Yield Point
The transition from elastic to plastic deformation is commonly called the yield point. Some stress–strain curves show a well‐recognized bump in this transition region which is taken as the yield point ( Figure 4.3a). On the other hand, it is often difficult to locate the yield point in some stress–strain curves because they change gradually in this region ( Figure 4.3b). When difficult to locate, the yield point is often taken at what is called an offset strain, equal to 0.2% strain. A straight line is drawn from the strain axis at 0.2%, parallel to the line for elastic deformation, and where this line crosses the stress–strain curve is taken as the yield stress.
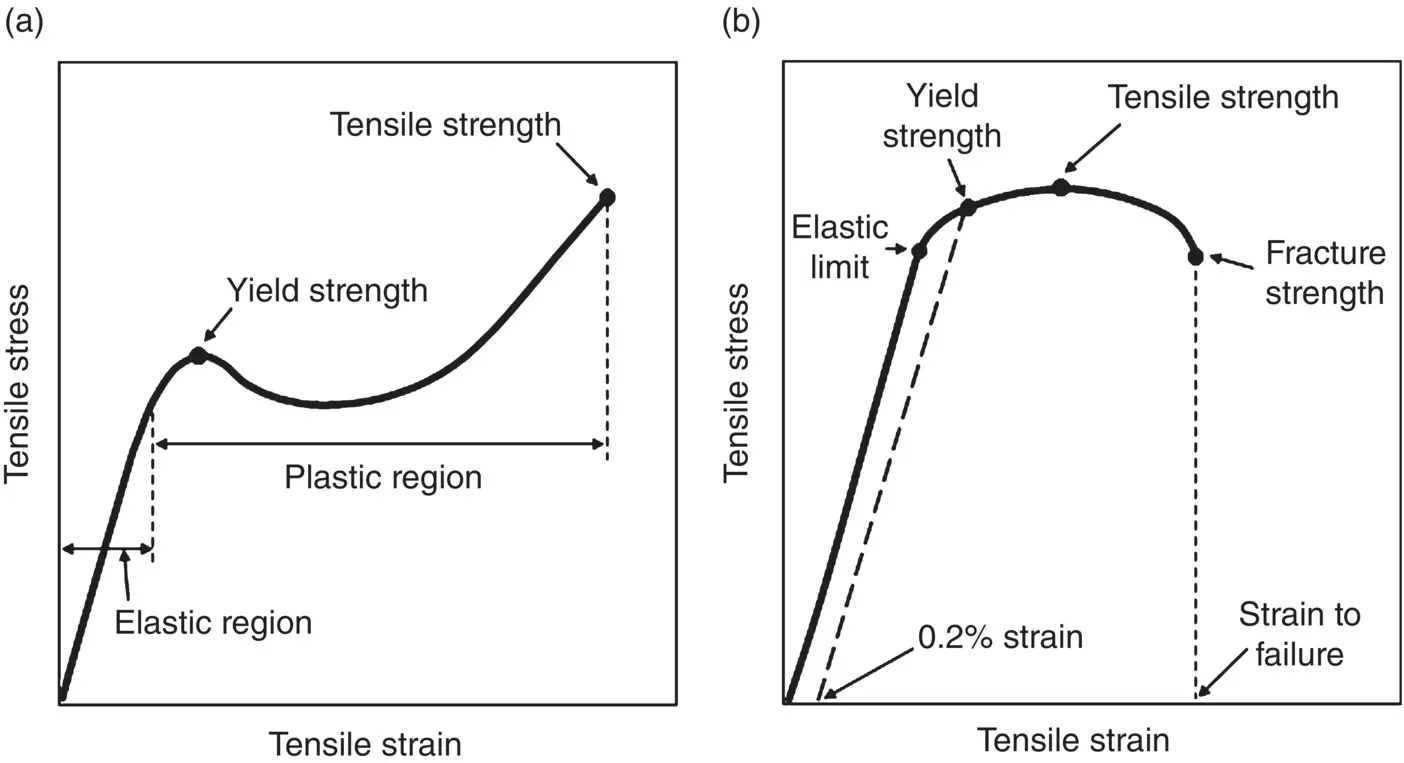
Figure 4.3 Schematic diagrams to illustrate two types of plastic deformation and yield behavior of ductile materials.
Definition and Determination of Strength
Various types of strength are used in the literature and, thus, it is useful to understand their definitions. As strength is determined from data obtained in various loading modes and is different in each loading mode, the specific type of strength should be stated when appropriate, such as tensile, compressive, shear, or flexural strength. In any loading mode, the strength is the highest stress that the material can support, sometimes referred to as the ultimate strength. The fracture strength (failure strength) is the stress at which the material fractures into two or more pieces. In tension or compression, strength is determined by taking the measured load and dividing it by the appropriate cross‐sectional area of the specimen. In torsion, the measured torque and specimen radius at failure along with Eqs. (4.8)and (4.9)are used, whereas in flexure, the measured load and the initial dimensions of the beam‐shaped specimen are substituted in Eq. (4.11)or Eq. (4.13). Commonly, several nominally identical specimens are tested and the strength is expressed as an average value plus or minus its standard deviation.
For ductile materials, such as metals, an important type of strength is the tensile yield strength, that is, the tensile stress at the yield point. Another type of strength that is often used is the tensile strength, sometimes called the ultimate tensile strength. Depending on the nature of the stress–strain curve, the tensile strength can be equal to or different from the fracture strength, that is, the strength at failure ( Figure 4.3). If the fracture strength is the highest stress in the curve, for example, the tensile strength and the fracture strength are the same.
True Stress and Strain Versus Engineering (Nominal) Stress and Strain
Читать дальше