When radiation causes the ejection of an electron from an atom of the absorber, the resulting positively charged atom and free negatively charged electron are called an ion pair( Figure 2.9). The amount of energy transferred per ion pair created, W, is characteristic of the materials in the absorber. For example, approximately 33 eV (range 25 to 40 eV) is transferred to the absorber for each ion pair created in air or water. It is often convenient to refer to the number of ion pairs created per unit distance the radiation travels as its specific ionization (SI).
Figure 2.8 Excitation and de‐excitation.
Figure 2.9 Ionization. The ejected electron and the positively charged atom are called an “ion pair” (demarcated by dashed line).
Particles with more charge (alpha particles) have a higher specific ionization than lighter particles (electrons).
Linear energy transfer (LET)is the amount of energy transferred in a given distance by a particle moving through an absorber. Linear energy transfer is related to specific ionization:
Alpha particles are classified as high LET radiation, beta particles and photons as low LET radiation.
Range is the distance radiation travels through the absorber. Particles that are lighter, have less charge (such as beta particles), and/or have greater energy travel farther than particles that are heavier, have a greater charge (such as alpha particles), and/or have less energy ( Figure 2.10).
In traversing an absorber, an electron loses energy at each interaction with the atoms of the absorber. The energy loss per interaction is variable. Therefore, the total distance traveled by electrons of the same energy can vary by as much as 3% to 4%. This variation in range is called the straggling of the ranges. The heavier alpha particles are not affected to a significant degree and demonstrate very little straggling of range.
This interaction in matter most often involves a positron (positive electron) and an electron (negatron). After a positron has transferred most of its kinetic energy by ionization and excitation, it combines with a free or loosely bound negative electron. Recall that electrons and positrons have equal mass but opposite electric charge. This interaction is explosive, as the combined mass of the two particles is instantly converted to energy in the form of two oppositely directed photons, each of 511 keV. This is referred to as an annihilation reaction( Figure 2.11). It is another example of the interchangeability of mass and energy described in Einstein’s equation: energy equals mass times the speed of light squared, or E = mc 2( Figure 2.12).
Small charged particles such as beta particles (electrons and positrons) may be deflected by nuclei as they pass through matter, which may be attributed to the positive charge of the atomic nuclei. As the electron or positron is deflected it also loses speed (decelerates) and this interaction generates X‐radiation known as bremsstrahlung ( Figure 2.13), which in German means “braking radiation.” The energy of the X‐ray is equal to the loss of the kinetic energy of the incident electron or proton as it decelerates (see section on kinetic energy). The greater the speed of the incident particle, the greater the charge of the nucleus, and the closer the incident particle is to the nucleus the greater the energy of the generated X‐ray ( Figure 2.14). Since the proximity of the incident particle to the nucleus is random, the X‐rays produced by a stream of particles will have a range of energies from near zero up to the kinetic energy of the incident particle (the maximum value is generated when the incident particle is completely stopped by the atomic nucleus). Figure 2.15illustrates the bremsstrahlung X‐ray spectrum for 90Yttrium which has a maximum X‐ray energy equal to the maximum beta particle energy of 2280 keV.
Figure 2.10 Particle range in an absorber.
Figure 2.11 Annihilation reaction.
Figure 2.12 Einstein’s theory of the equivalence of energy and mass.
Figure 2.13 Bremsstrahlung. Beta particles (β –) and positrons (β +) that travel near the nucleus will be attracted or repelled by the positive charge of the nucleus generating X‐rays in the process.
1 1. Shapiro, J. Radiation Protection. A Guide for Scientists, Regulators, and Physicians, 4th edn. Cambridge MA: Harvard University Press, 2002, pp. 42 and 53.
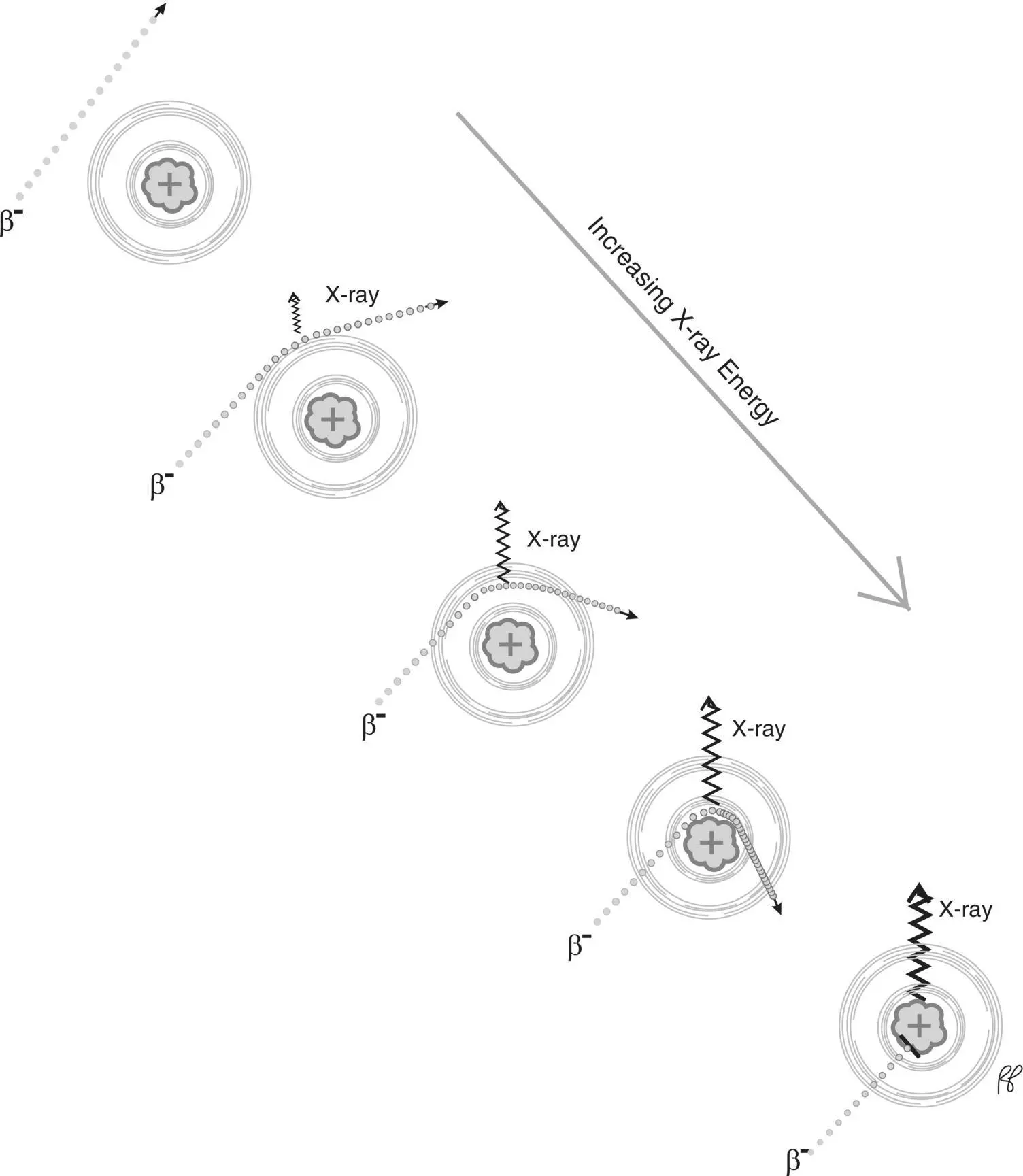
Figure 2.14 Bremsstrahlung X‐ray energies increase with increasing proximity of the beta particle to the atomic nucleus (illustrated), greater incident beta particle energy, and greater nucleus charge.
Figure 2.15 Bremsstrahlung X‐ray energies vary from near zero to the maximum incident beta particle energy.
1 Which of the following is true of the interaction of charged particles with matter?Alpha particles have a higher LET than beta particles.The range of alpha particles is generally greater than beta particles.Alpha particles have a higher specific ionization than beta particles.
2 True or false: Bremsstrahlung is X‐ray radiation emitted as a moving electron or positron decelerates and changes direction in close proximity to a nucleus.
3 True or false: The photoelectric effect is the dominant type of photon interaction in tissue for radionuclides used in the practice of nuclear medicine.
4 For each of the terms listed here, select the appropriate definition:HVL (half value layer).TVL (tenth value layer).μ (mu) (linear attenuation coefficient).Thickness of an attenuator that will reduce the intensity (number of photons) in a monoenergetic beam by 90%.Thickness of an attenuator that will reduce the intensity (number of photons) in a monoenergetic beam by 50%.0.693/HVL.
Читать дальше