Nirmal K. Sinha - Engineering Physics of High-Temperature Materials
Здесь есть возможность читать онлайн «Nirmal K. Sinha - Engineering Physics of High-Temperature Materials» — ознакомительный отрывок электронной книги совершенно бесплатно, а после прочтения отрывка купить полную версию. В некоторых случаях можно слушать аудио, скачать через торрент в формате fb2 и присутствует краткое содержание. Жанр: unrecognised, на английском языке. Описание произведения, (предисловие) а так же отзывы посетителей доступны на портале библиотеки ЛибКат.
- Название:Engineering Physics of High-Temperature Materials
- Автор:
- Жанр:
- Год:неизвестен
- ISBN:нет данных
- Рейтинг книги:3 / 5. Голосов: 1
-
Избранное:Добавить в избранное
- Отзывы:
-
Ваша оценка:
- 60
- 1
- 2
- 3
- 4
- 5
Engineering Physics of High-Temperature Materials: краткое содержание, описание и аннотация
Предлагаем к чтению аннотацию, описание, краткое содержание или предисловие (зависит от того, что написал сам автор книги «Engineering Physics of High-Temperature Materials»). Если вы не нашли необходимую информацию о книге — напишите в комментариях, мы постараемся отыскать её.
Discover a comprehensive exploration of high temperature materials written by leading materials scientists Engineering Physics of High-Temperature Materials: Metals, Ice, Rocks, and Ceramics
Engineering Physics of High-Temperature Materials (EPHTM)
Engineering Physics of High-Temperature Materials
Engineering Physics of High-Temperature Materials: Metals, Ice, Rocks, and Ceramics
Engineering Physics of High-Temperature Materials — читать онлайн ознакомительный отрывок
Ниже представлен текст книги, разбитый по страницам. Система сохранения места последней прочитанной страницы, позволяет с удобством читать онлайн бесплатно книгу «Engineering Physics of High-Temperature Materials», без необходимости каждый раз заново искать на чём Вы остановились. Поставьте закладку, и сможете в любой момент перейти на страницу, на которой закончили чтение.
Интервал:
Закладка:
It should be recognized that ice engineering is part of a new engineering frontier – the frontier of engineering physics of high‐temperature material science and application. Like any other high‐temperature material, ice also exhibits certain unavoidable complexities. “Delayed elastic” response, the essential element of the EDEV theory presented in this book, is strictly a high‐temperature phenomenon (Sinha 1979). Time‐dependent elastic or “delayed elastic” or “anelastic” (not a preferred term according to British Standard Institution 1975) behavior is a high‐temperature material response and it is demonstrated herein that this response leads to the explanations of complex and wide‐ranging issues for materials science and engineering applications, including geophysics of post‐glacial rebound ( Chapter 10) and plate tectonics ( Chapter 11).
1.3.2 Ice: An Analog to Understand High‐Temperature Properties of Solids
Taking a multidisciplinary approach using research in various fields enables an integrated cross‐disciplinary viewpoint that removes barriers of understanding across and within different fields.
Think of an ice cube floating at the top in a glass of drinking water in your hand. That ice cube may contain hundreds of crystals and the water is the liquid state of ice. Similarly, a floating ice cover in rivers, lakes, and oceans is a solid plate floating on its own melt. The bottom surface of an ice cover is always at its melting point, T m, and temperatures at the upper surfaces are not far from T m. The complexities that we see happening in ice sheets in “short observable times” (seconds, minutes, or few hours) occur under our feet over millennia or millions of years. As mentioned earlier, the correspondence between geophysical aspects of tectonics and “floating ice sheets” is striking as an analog model material. Wind driven snow cover on a floating ice sheet is like the lithosphere on ice that is not unlike the asthenosphere above the water, equivalent to the lower part of the mantle. An ice sheet bends, creeps, and cracks when a load is placed on it (as described in detail in Chapter 10). When ice floes are driven by the wind and/or the undercurrent, they react with each other, pile up, and form ridges, very similar to the formation of mountains and continents caused by plate tectonic forces. Ridge formations in ice may take a few seconds or minutes, but millions of years are taken to build up mountains due to plate tectonics. How can one reconcile these two apparently different phenomena? An effort for reconciliation is made in Chapters 10and 11. As incredible as it may sound, it will be shown that an event lasting for 1 s at say 0.98 T mcould be equivalent to several million years at 0.2 T m.
Generally, materials science experimentalists and theoreticians promote the “steady‐state” aspects of deformation of materials at high temperatures. Not surprisingly, it is extended to mantle physics. Similarly, glaciologists (working only on flow of glaciers) are concerned singularly with “steady‐state” creep response. Geophysicists are certainly aware of the role of transient creep of rocks largely because of earthquake‐related problems. However, other than dislocation creep, they have largely dealt with phenomenological aspects of rheology, not necessary from the micromechanical point of view. Now, there is a growing interest among geophysicists to focus on the transient creep of geologic materials (Karato 1998; Birger 2016). However, effects of grain size on transient creep and closely related delayed elastic response of rocks at high temperatures are still to be recognized and explored.
Geologists, geophysicists, and metallurgists do not necessarily have to deal with high‐temperature issues as much as ice engineers and glaciologists have to, simply because ice always exists at temperatures very close to its melting point. Most ice engineering problems involve extremely short‐term response well within the transient creep (Gold and Sinha 1980). Engineers dealing with ice engineering in Canada, the Northern States, Scandinavia, Russia, and China were thus baffled for a long time. No wonder, ice engineers facing complex mechanical responses of ice used to conclude, “ice is a peculiar material” – for example, its Young's modulus “depends” on grain size and changes with time and loading rates.
It may sound ludicrous; however, seawater (containing unconsolidated frazil crystals) may be considered as one of the simplest analogs of low‐viscosity magma containing suspended crystals and floating sea ice with snow covers equivalent to the asthenosphere and lithosphere, respectively. Often, the layers of low‐viscosity frazil‐rich seawater reach a great depth, enough to hinder maneuvering of ice‐strengthened ships, including nuclear‐powered Russian icebreakers. As the viscosity of seawater increases with decrease in temperature, constitutional supercooling occurs and pockets of concentrated brine are trapped within the ice mass. With further decrease in temperature, different types of crystals form within the pockets depending on their eutectic points, as illustrated in Figure 1.3.
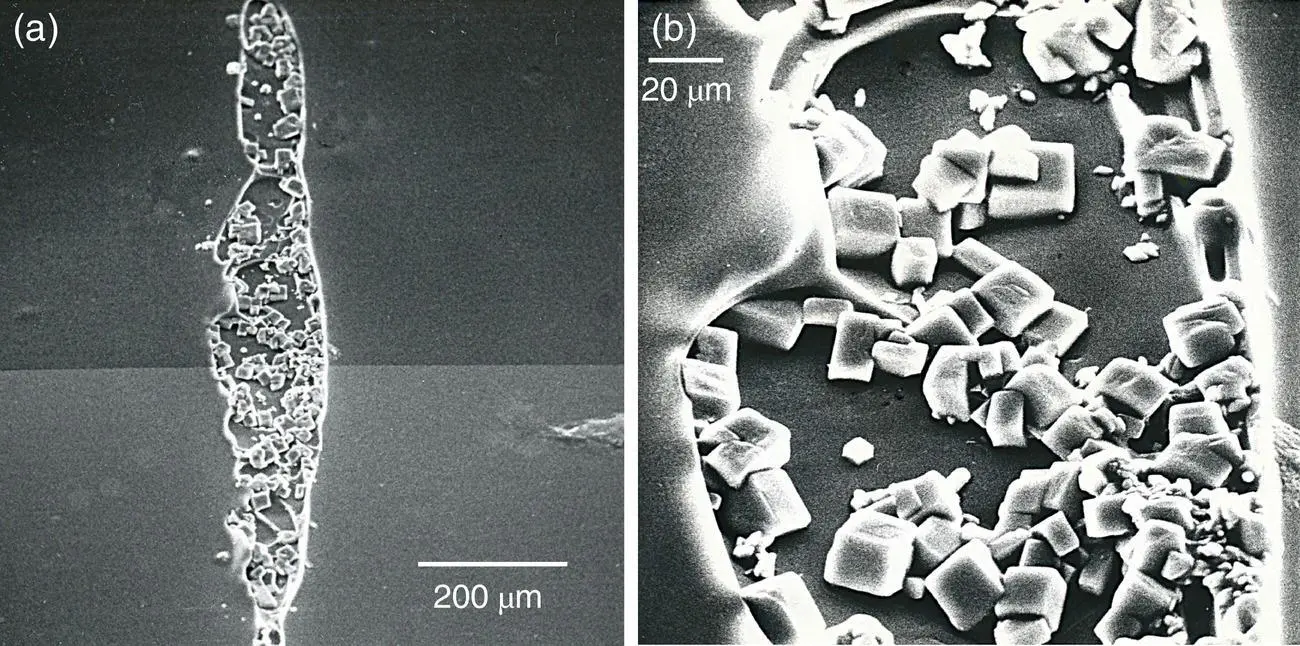
Figure 1.3 Scanning electron micrograph of (a) a vertical section of a brine pocket at −30 °C in columnar‐grained first‐year sea ice with salinity of 4‰, and (b) details of precipitated salt crystals.
Source: Modified from Sinha (1977).
Even though water is the basis of life on Earth and has been the subject of the most scientific scrutiny for several centuries, the solid state of water – or ice – has rarely been considered as a “hot crystalline material.” Glaciologists may be considered as the pioneers in examining ice as a solid crystalline material for over a century, but they tend to consider glacier ice as “cold” and the subject of glaciology as special. Viscoelastic flow properties of glacier ice were formulated during the 1950s and are well known. Rarely, however, has a glacier been compared to a bed or river of molten rock or lava flowing viscoelastically (not necessarily linear viscoelastic) down the mountain side. High‐temperature creep deformation in many cases, like flow in glaciers, involves syntectonic (geology describing any process that accompanies a tectonic one) fabric development, yet this critical topic is not mentioned in the field of glaciology as an elevated temperature phenomenon. Only recently has effort been made in establishing one‐to‐one correspondence on a physical basis between the cracking and flowing characteristics in glaciers and those in semisolid lava or igneous rock undergoing complex high‐temperature flow as well.
Of course, some peculiar effects take place in glaciers that are different during the flow of lava. This has to do with change in density of water on solidification. The melting point of most crystalline materials on Earth and metals and metallic alloys increase with increase in pressure. This is due to the increase in density on solidification unlike water changing its phase to ice. Naturally, applications of general rheological flow to glaciology must give consideration to pressure effects in the case of ice. However, such effects have not been completely sorted out within the glaciology field so it would be inappropriate to claim that the proposed ideas would somehow offer a unifying model applicable to all aspects of glaciology.
High‐temperature mechanical properties of materials are characterized by their creep life. Creep and fracture properties are governed by structural changes, as well as damages in the form of cavities and cracks that occur during deformation. Most tests are performed in air. It is convenient to perform tests in air, simply because providing an inert environment during tests is very difficult. However, oxidation starting from the exposed surfaces of specimens in pure metals and alloys at elevated temperatures is unavoidable. Materials are degraded or damaged by the formation of voids preferably along the grain boundaries (being at higher energy states). For example, Wilshire and Battenbough (2007) performed tensile creep and rupture tests on fine‐grained (≈40 μm) polycrystalline pure copper under truly constant stress (using the machine described in Evans and Wilshire 1985), as well as constant load at 686–823 K (about 0.5–0.6 T m), and demonstrated an increasing number of voids with increasing distance from the surface. They reported that fracture invariably occurs by cavitation, with the following observations noted: (i) Isolated grain‐boundary cavities are evident late in the primary stage during creep in both the n ∼ = 1 and n ∼ = 4.5 regimes, (ii) cavities form preferentially near specimen surfaces, with the numbers of cavities and cracks decreasing from the surface to the center due primarily to the formation of oxide particles created by oxygen ingress along grain boundaries during creep exposure (Parker and Wilshire 1980), and (iii) the incidence of cavities increases with increasing creep strain, eventually forming single‐grain‐facet cracks that are linked to producing large multigrain‐facet cracks at various locations along the gauge length of fractured specimens.
Читать дальшеИнтервал:
Закладка:
Похожие книги на «Engineering Physics of High-Temperature Materials»
Представляем Вашему вниманию похожие книги на «Engineering Physics of High-Temperature Materials» списком для выбора. Мы отобрали схожую по названию и смыслу литературу в надежде предоставить читателям больше вариантов отыскать новые, интересные, ещё непрочитанные произведения.
Обсуждение, отзывы о книге «Engineering Physics of High-Temperature Materials» и просто собственные мнения читателей. Оставьте ваши комментарии, напишите, что Вы думаете о произведении, его смысле или главных героях. Укажите что конкретно понравилось, а что нет, и почему Вы так считаете.