This complex alternative process is crucial to the survival of pathogenic intestinal bacteria. Pathogens can use ethanolamine, but only when vitamin B 12is present. Since eut genes are not present in commensal bacteria, they provide pathogens a significant advantage over commensal bacteria.
RNAIII in Staphylococcus aureus
The RNAIII of S. aureus is regulated by quorum sensing , which means it is expressed once the bacterial population reaches a certain threshold. RNAIII controls the expression of a certain number of virulence factors. It impedes the translation of proteins, such as protein A, expressed on the surface of the bacterium or secreted during the beginning of an infection. It also impedes the translation of transcription regulators such as RotA. However, it activates the expression of the toxin known as alpha-hemolysin (Hla) by acting as an antisense that allows the corresponding RNA to be translated. Additionally, RNAIII codes for the small Hld protein, another toxin of 26 amino acids. The 514-nucleotide-long RNAIII in S. aureus is a very active molecule and thus can regulate many facets of bacterial physiology over the course of an infection.
Some RNAs function both as antisense and as messenger. They are encoded in recently discovered regions of bacterial chromosomes called excludons . These regions were originally detected in the Listeria genome but were then found to exist in various other bacteria. Excludons are made up of two DNA regions encoding genes or operons that are oriented in opposition to one another on the bacterial chromosome. They encode a long RNA (up to 6,000 nucleotides) that is antisense to one of the regions. The first part of this RNA functions as an actual antisense that has a negative effect on the genetic expression of the gene or operon located on the strand opposite to the one that codes the RNA. But the second part of the RNA can act as an mRNA ( Fig. 8).
Figure 8. Example of an excludon. Once the transcription beginning at P2 is expressed and generates a long transcript, the operon on the right becomes less expressed.
CHAPTER 4
From the CRISPR Defense System to the CRISPR/ Cas9 Method for Modifying Genomes
In nature, bacteria need to defend themselves constantly, particularly against bacteriophages (or phages), the viruses that specifically attack bacteria. A phage generally attaches itself to a bacterium, injects its DNA into it, and subverts the bacterium’s mechanisms of replication, transcription, and translation in order to replicate itself. The phage DNA reproduces its own DNA, transcribes it into RNA, and produces phage proteins that accumulate to generate new phages and eventually cause the bacterial cell to explode (or lyse), releasing hundreds of new bacteriophages. Phages continually infect bacteria everywhere—in soil, in water, and even in our own intestinal microbiota ( Fig. 9). Bacteriophage families are numerous and vary widely in their form, size, composition, and the bacteria they target.
To begin their attack, bacteriophages need a site of attachment, a particular component on the surface of a bacterium. This site of attachment is specific for each virus and the bacteria that it can infect.
Infections of bacteria by phages are of great concern, particularly in the dairy industry, which uses certain bacteria, for example Streptococcus thermophilus , to make yogurt and cheese. S. thermophilus transforms the lactose in milk into lactic acid. Additionally, each different bacterial strain contributes its own unique taste and texture to the yogurt, which must remain consistent to ensure a reliable product and successful sales. If a bacterial strain disappears as the result of a bacteriophage infection, the economic consequences for the manufacturer can be disastrous.
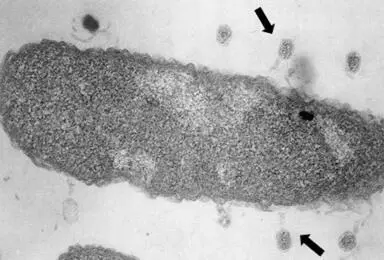
Figure 9. Bacteriophages infecting an Escherichia coli bacterium.
One of the great discoveries of this decade is that bacteria have an immune system called CRISPR , for clustered regularly interspaced short palindromic repeats, meaning small, regularly spaced, palindromic (the sequence reads the same from either end) repeats. CRISPR regions in the chromosomes allow bacteria to recognize predators, particularly previously encountered phages, and to destroy them. CRISPR regions protect and essentially “vaccinate” bacteria against bacteriophages.
In fact, it has been shown that bacteria can be artificially vaccinated! When a population of bacteria is inoculated with a phage, a small number survive and are able to integrate a fragment of the phage DNA into their genome, in the region called the CRISPR locus. This allows the bacteria, if the phage ever attacks again, to recognize the phage DNA and degrade it. This ingenious phenomenon, known as interference , occurs due to the structure of the CRISPR region and to cas genes ( C RISPR- as sociated genes) located near this region.
The CRISPR locus is a region of the chromosome composed of repeated sequences of around 50 nucleotides, interspersed with sequences known as spacers that are similar to those of bacteriophages. Some bacteria have several CRISPR loci with different sequence repetitions. Around 40% of bacteria have one or more CRISPRs, whereas others have none. CRISPR loci can be quite long, sometimes with more than 100 repetitions and spacers. CRISPRs have two functions: acquisition and interference. Acquisition , also called adaptation , is the process of acquiring fragments of DNA from a phage, and interference is the immunization process by Cas proteins encoded by cas genes ( Fig. 10).
Bacteria have numerous proteins with various complementary and synergistic functions in the process of adaptation and interference. They permit the addition of DNA fragments into the CRISPR locus, but their main purpose is to react to invading phages. The CRISPR locus is transcribed into a long CRISPR RNA, which is then split into small RNAs called crRNAs, each containing a spacer and a part of the repeated sequence. When a phage injects its DNA into the bacterium, the crRNA recognizes and binds to it. An enzyme then recognizes the hybrid and cleaves the phage DNA at the point where the crRNA has paired. Replication of the phage DNA is inactivated, and the infection is stopped.
One of the key discoveries that led to the use of CRISPR systems in what is called “genome editing,” or modification , was the identification of the proteins involved in the cleavage of the hybrid DNA. This process is performed by a complex of proteins containing the protein Cas1 and sometimes by a single protein called Cas9. Cas9 is unique in that it can attach itself to a DNA strand and, due to the two distinct domains of its structure, cut this DNA on each of its two strands. This protein is the basis of the CRISPR/Cas9 technology, which enables a variety of genome modifications and mutations in mammals, plants, insects, and fish in addition to bacteria. This system works due to the Cas9 protein and also a guide RNA hybrid that is made from one RNA similar to the region to be mutated and a second RNA called tracrRNA , or trans-activating crRNA. tracrRNA was discovered next to the CRISPR locus in Streptococcus pyogenes and was shown to be homologous to the repeated regions of the locus, enabling it to guide the Cas9 protein and the crRNA toward the target.
Читать дальше