The discussion so far has concentrated on periods with IMF B Z< 0 when reconnection occurs at the subsolar magnetopause. When the IMF turns northward, the region of high magnetic shear on the magnetopause moves to high latitudes, where both closed and open field lines are available to reconnect. Figure 2.13shows the reconnection topologies that can ensue (Cowley, 1981a). Panels (d) and (e) show reconnection in only one hemisphere with a closed or open field line. Panels (a) and (b) show reconnection in both hemispheres with closed field lines. Finally, panel (c) shows reconnection occurring with open field lines in both hemispheres. Panels on the right show the magnetosphere looking from the Sun, with impinging IMF field lines with B Z> 0 and B Y< 0 (Imber et al., 2006). In (A), the B Ycomponent is such that separate IMF field lines reconnect at the northern and southern reconnection sites, corresponding to panels (d) and (e). Panel (B) shows the limiting clock angle for which a single IMF field line can reconnect at both north and south, corresponding to panels (a) to (c).
Figure 2.13 (Left) The topology of field lines that can undergo reconnection when IMF B Z> 0 (from Cowley, 1981). (Right) Single lobe reconnection and the limiting angle for dual lobe reconnection
(adapted from Cowley, 1981; Imber et al., 2006).
If reconnection takes place with closed field lines, the Dungey cycle operates as for southward IMF, but east‐west asymmetries in the dayside flows will be significant. On the other hand, if reconnection occurs with lobe field lines, convection of a different nature is excited. The situations in (c) and (e) are referred to as “dual‐” and “single‐lobe reconnection” (DLR and SLR), respectively. Reasonable estimates of the lengths of the lobe reconnection X‐lines suggest that the limiting clock angle for DLR is | θ | ≲ 10° (Imber et al., 2006). Figure 2.14contrasts the convection expected for southward IMF, SLR, and DLR. In SLR, the lobe reconnection site now maps inside the polar cap ionosphere on open field lines. Newly reconnected field lines are pulled initially sunward by magnetic tension forces (i.e., Fig. 2.13e), before being swept tailward by the flow of the solar wind. The result is a circulation in the polar cap in the form of two reverse lobe cells ( Fig. 2.14b and top middle panel of Fig. 2.1). For nonzero IMF B Y, one or other of the cells enlarges (e.g., Huang et al., 2000). The vorticity of the lobe cells is associated with upward and downward FAC in the dayside polar cap, known as NBZ (northward B Z) currents, and the upward FAC associated with the clockwise‐rotating cell produces auroral emission (e.g., Frey et al., 2004; Carter et al., 2018). A “cusp auroral spot” can also be formed by direct precipitation of magnetosheath plasma along the newly reconnected field lines, that is in the sunward flow region of the reverse cells (Milan et al., 2000b). No net flux is opened or closed by SLR, and the polar cap remains of uniform size. In the case of DLR, open field lines are closed and the polar cap contracts as the lobes are eroded, with sunward flow out of the polar cap at noon ( Fig. 2.14c) (Imber et al., 2006).
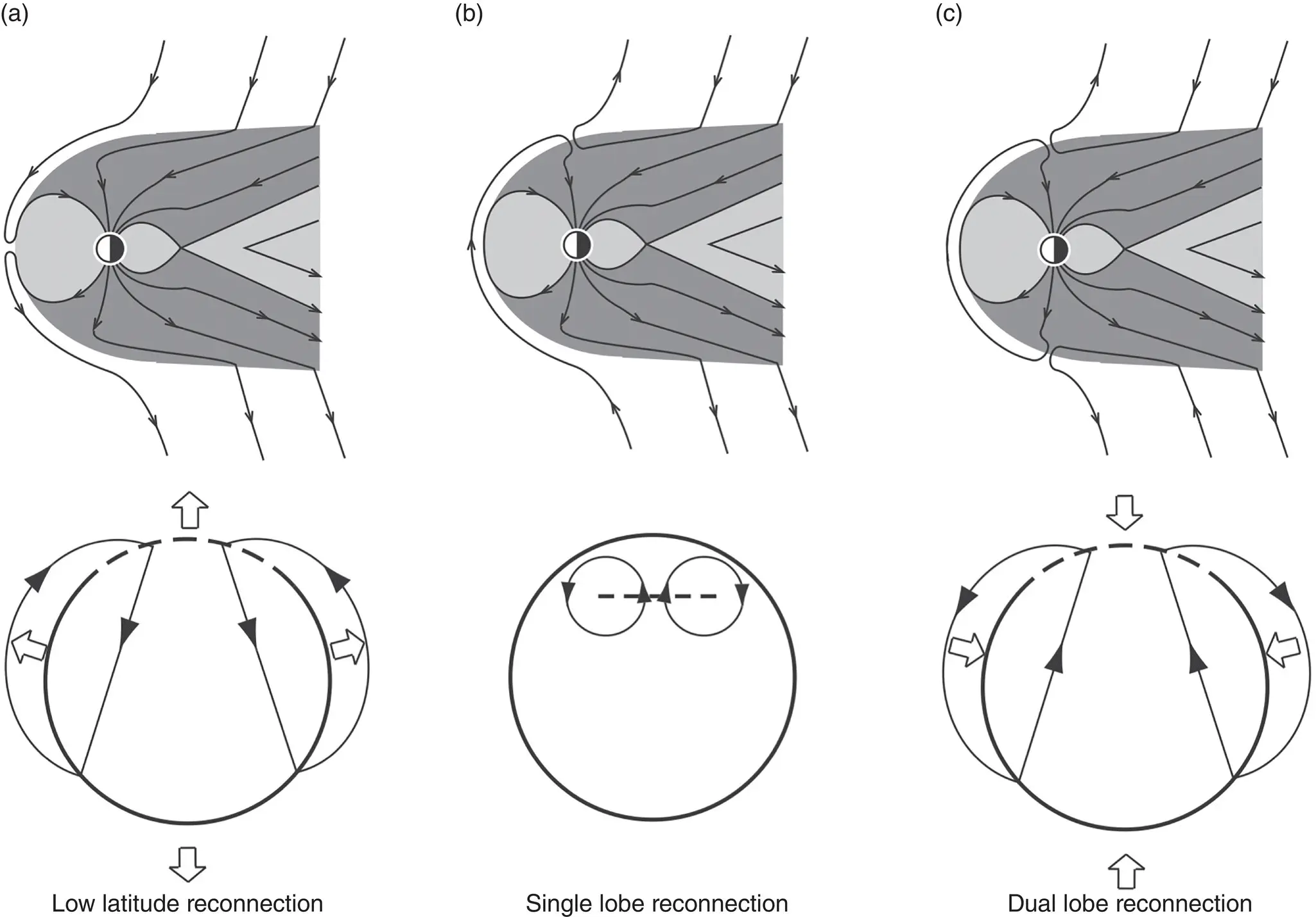
Figure 2.14 Contrasting the convection patterns for southwards IMF, and for single‐ and dual‐lobe reconnection occurring for northward IMF
(from Imber et al., 2006; https://www.ann‐geophys.net/24/3115/2006/. Licensed under CC BY 3.0).
The lobe cells in the top middle panel of Figure 2.1appear to be embedded within weak twin‐cell convection. Some studies ascribe this to a viscous interaction between the flow of the solar wind and the magnetopause, producing antisunward flows in the flanks of the magnetosphere, with return flow deeper inside, as first proposed by Axford and Hines (1961). In the ionosphere, this would produce two convection cells with the same sense of vorticity as the Dungey cells, but entirely in the closed field line regions to the dawn and dusk of the polar cap. If a viscous interaction and the Dungey cycle operated together, these new cells would be embedded within the Dungey cells, and displace the dawn and dusk convection reversals equatorward of the polar cap boundary (e.g., Reiff & Burch, 1985). The role of a viscous interaction is still debated, though there is yet to be definitive evidence of viscous cells within the ionospheric convection pattern. Moreover, it has been suggested that the appearance of twin‐cell convection during periods when the Dungey cycle is not expected to be active is caused by residual tail reconnection still ongoing from substorm activity initiated during previous periods of southward IMF (e.g., Milan, 2004), or associated with TRINNIs. There is evidence to suggest that the reverse cells also become more apparent after the IMF has been northward for more extended periods of time (Grocott & Milan, 2014), possibly as a result of the preexisting nightside reconnection‐driven flows subsiding.
This review has discussed the excitation of ionospheric convection by solar wind‐magnetosphere coupling, and the role of magnetospheric currents in magnetosphere‐ionosphere coupling. We have focused on large‐scale dynamics, in an attempt to provide a holistic view of the coupled system. Further details can be found in the reviews cited in the introduction. There are features and processes that have not been covered in this review, but which are discussed in other chapters of this monograph. The energy input to the high‐latitude ionosphere through the electrodynamics discussed here is described in Chapter 1; large‐ and small‐scale polar cap and midlatitude dynamics during substorms and geomagnetic storms are covered in Chapters 3, 7, and 15; the ionospheric plasma structure produced by convection is the topic of Chapter 4. In addition, processes described in this review, including ionospheric heating, can produce significant outflow of ions from the ionosphere into the magnetosphere, with implications for subsequent magnetospheric dynamics, as discussed in Chapter 5. Another feature that has been neglected in this review is the formation of rapid westward convection flows in the duskside subauroral ionosphere, known as subauroral polarization streams (SAPS) and associated with the inner magnetosphere plasma dynamics; this is covered in detail in Chapter 6. Finally, we have not discussed the role of interhemispheric asymmetries associated with dipole tilt, seasonal variations in ionospheric illumination, and the influence of the B Xcomponent of the IMF, and these are described in Chapter 8.
SEM was supported by STFC grant ST/N000749/1. AG was supported by NERC grant NE/P001556/1 and STFC grant ST/R000816/1.
1 Acuña, M. H., Ogilvie, K. W., Baker, D. N., Curtis, S. A., Fairfield, D. H., & Mish, W. H. (1997). The Global Geospace Science Program and its investigations. Space Science Reviews, 71, 5. doi:10.1007/BF00751323
2 Akasofu, S.‐I. (1964). The development of the auroral substorm. Planetary and Space Science, 12, 273–282. doi: 10.1016/0032‐0633(64)90151‐5
3 Alfvén, H. (1942). Existence of electromagnetic‐hydrodynamic waves. Nature, 150, 405. doi: 10.1038/150405d0
Читать дальше