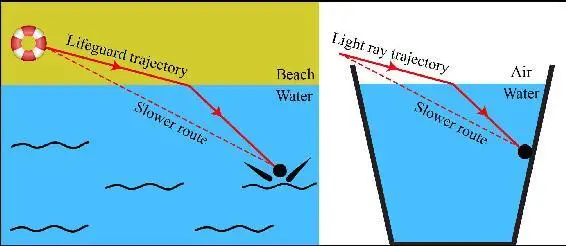
Figure 7.1: To rescue a swimmer as fast as possible, a lifeguard won’t go in a straight line (dashed), but a bit further along the beach where she can go faster than in the water. A light ray similarly bends when entering the water to reach its destination as fast as possible.
One famous quantity that nature strives to maximize is entropy, which loosely speaking measures how messy things are. The second law of thermodynamics states that entropy tends to increase until it reaches its maximum possible value. Ignoring the effects of gravity for now, this maximally messy end state is called heat death, and corresponds to everything being spread out in boring perfect uniformity, with no complexity, no life and no change. When you pour cold milk into hot coffee, for example, your beverage appears to march irreversibly toward its own personal heat death goal, and before long, it’s all just a uniform lukewarm mixture. If a living organism dies, its entropy also starts to rise, and before long, the arrangement of its particles tends to get much less organized.
Nature’s apparent goal to increase entropy helps explain why time seems to have a preferred direction, making movies look unrealistic if played backward: if you drop a glass of wine, you expect it to shatter against the floor and increase global messiness (entropy). If you then saw it unshatter and come flying back up to your hand intact (decreasing entropy), you probably wouldn’t drink it, figuring you’d already had a glass too many.
When I first learned about our inexorable progression toward heat death, I found it rather depressing, and I wasn’t alone: thermodynamics pioneer Lord Kelvin wrote in 1841 that “the result would inevitably be a state of universal rest and death,” and it’s hard to find solace in the idea that nature’s long-term goal is to maximize death and destruction. However, more recent discoveries have shown that things aren’t quite that bad. First of all, gravity behaves differently from all other forces and strives to make our Universe not more uniform and boring but more clumpy and interesting. Gravity therefore transformed our boring early Universe, which was almost perfectly uniform, into today’s clumpy and beautifully complex cosmos, teeming with galaxies, stars and planets. Thanks to gravity, there’s now a wide range of temperatures allowing life to thrive by combining hot and cold: we live on a comfortably warm planet absorbing 6 , 000°C (10 , 000°F) solar heat while cooling off by radiating waste heat into frigid space whose temperature is just 3°C (5°F) above absolute zero.
Second, recent work by my MIT colleague Jeremy England and others has brought more good news, showing that thermodynamics also endows nature with a goal more inspiring than heat death.1 This goal goes by the geeky name dissipation-driven adaptation, which basically means that random groups of particles strive to organize themselves so as to extract energy from their environment as efficiently as possible (“dissipation” means causing entropy to increase, typically by turning useful energy into heat, often while doing useful work in the process). For example, a bunch of molecules exposed to sunlight would over time tend to arrange themselves to get better and better at absorbing sunlight. In other words, nature appears to have a built-in goal of producing self-organizing systems that are increasingly complex and lifelike, and this goal is hardwired into the very laws of physics.
How can we reconcile this cosmic drive toward life with the cosmic drive toward heat death? The answer can be found in the famous 1944 book What’s Life? by Erwin Schrödinger, one of the founders of quantum mechanics. Schrödinger pointed out that a hallmark of a living system is that it maintains or reduces its entropy by increasing the entropy around it. In other words, the second law of thermodynamics has a life loophole: although the total entropy must increase, it’s allowed to decrease in some places as long as it increases even more elsewhere. So life maintains or increases its complexity by making its environment messier.
Biology: The Evolution of Goals
We just saw how the origin of goal-oriented behavior can be traced all the way back to the laws of physics, which appear to endow particles with the goal of arranging themselves so as to extract energy from their environment as efficiently as possible. A great way for a particle arrangement to further this goal is to make copies of itself, to produce more energy absorbers. There are many known examples of such emergent self-replication: for example, vortices in turbulent fluids can make copies of themselves, and clusters of microspheres can coax nearby spheres into forming identical clusters. At some point, a particular arrangement of particles got so good at copying itself that it could do so almost indefinitely by extracting energy and raw materials from its environment. We call such a particle arrangement life . We still know very little about how life originated on Earth, but we know that primitive life forms were already here about 4 billion years ago.
If a life form copies itself and the copies do the same, then the total number will keep doubling at regular intervals until the population size bumps up against resource limitations or other problems. Repeated doubling soon produces huge numbers: if you start with one and double just three hundred times, you get a quantity exceeding the number of particles in our Universe. This means that not long after the first primitive life form appeared, huge quantities of matter had come alive. Sometimes the copying wasn’t perfect, so soon there were many different life forms trying to copy themselves, competing for the same finite resources. Darwinian evolution had begun.
If you had been quietly observing Earth around the time when life got started, you would have noticed a dramatic change in goal-oriented behavior. Whereas earlier, the particles seemed as though they were trying to increase average messiness in various ways, these newly ubiquitous self-copying patterns seemed to have a different goal: not dissipation but replication . Charles Darwin elegantly explained why: since the most efficient copiers outcompete and dominate the others, before long any random life form you look at will be highly optimized for the goal of replication.
How could the goal change from dissipation to replication when the laws of physics stayed the same? The answer is that the fundamental goal (dissipation) didn’t change, but led to a different instrumental goal , that is, a subgoal that helped accomplish the fundamental goal. Take eating, for example. We all seem to have the goal of satisfying our hunger cravings even though we know that evolution’s only fundamental goal is replication, not mastication. This is because eating aids replication: starving to death gets in the way of having kids. In the same way, replication aids dissipation, because a planet teeming with life is more efficient at dissipating energy. So in a sense, our cosmos invented life to help it approach heat death faster. If you pour sugar on your kitchen floor, it can in principle retain its useful chemical energy for years, but if ants show up, they’ll dissipate that energy in no time. Similarly, the petroleum reserves buried in the Earth’s crust would have retained their useful chemical energy for much longer had we bipedal life forms not pumped it up and burned it.
Among today’s evolved denizens of Earth, these instrumental goals seem to have taken on a life of their own: although evolution optimized them for the sole goal of replication, many spend much of their time not producing offspring but on activities such as sleeping, pursuing food, building homes, asserting dominance and fighting or helping others—sometimes even to an extent that reduces replication. Research in evolutionary psychology, economics and artificial intelligence has elegantly explained why. Some economists used to model people as rational agents, idealized decision makers who always choose whatever action is optimal in pursuit of their goal, but this is obviously unrealistic. In practice, these agents have what Nobel laureate and AI pioneer Herbert Simon termed “bounded rationality” because they have limited resources: the rationality of their decisions is limited by their available information, their available time to think and their available hardware with which to think. This means that when Darwinian evolution is optimizing an organism to attain a goal, the best it can do is implement an approximate algorithm that works reasonably well in the restricted context where the agent typically finds itself. Evolution has implemented replication optimization in precisely this way: rather than ask in every situation which action will maximize an organism’s number of successful offspring, it implements a hodgepodge of heuristic hacks: rules of thumb that usually work well. For most animals, these include sex drive, drinking when thirsty, eating when hungry and avoiding things that taste bad or hurt.
Читать дальше