Now, atoms actually have a finite diameter, of about 10 –8centimetres. So the ionization of an atom is not a perfect position measurement, and this has important consequences for the alpha-particle tracks. It is helpful to think in terms of the blue mist. Before the measuring ionization happens, the blue mist is expanding outwards uniformly in all directions. When the first ionization occurs, it is as if a spherical shell has suddenly been placed round the atom. At one point on the shell there is a small hole through which the wave function can pass. This is the point at which the ionized atom is situated. It is only here that the wave function is not totally destroyed and can continue streaming on outwards. In fact, it does so in the form of a jet, which can be very narrow and accurately directed, especially if the alpha particle has a high energy.
At this point it is worth saying something about the diffraction of light. If monochromatic light (light of one wavelength) encounters an opaque screen with an opening, the result depends on its size. If the opening is large compared with the wavelength of the light, the screen cuts off all the light except at the opening and a more or less perfect ‘pencil’ of light – a beam – passes through. The width of the luminous pencil is equal to the width of the opening. However, if the opening is made smaller, diffraction comes into play and the beam of light spreads out, becoming very diffuse for a tiny opening. Diffraction effects are more pronounced for red light, with its longer wavelength, than violet light. Like light, alpha particles have an associated wavelength, which is very short for the ones produced in radioactive decay. Although ionization of the atom creates effectively a very small ‘opening’, the ‘jet of wave function’ that survives the wave-function collapse is narrow and concentrated in a cone with a very small opening angle (much less than a degree). The wave-function jet continues through the cloud chamber like a searchlight beam.
To simplify things, imagine that the cloud-chamber atoms are concentrated on uniformly spaced, spherical concentric shells surrounding the radium atom. The first ionization (quantum measurement and collapse) happens when the alpha particle’s spherical waves reach the first shell. On the second shell, the alpha particle can ionize atoms only where its wave function has non-vanishing value. The atoms that can be ionized are located in the small spot that is ‘lit up’ by the ‘beam’ and hence lie rather accurately on the line joining the radium atom to the ‘opening’ in the first shell. The spot still contains many hundreds or thousands of atoms, any one of which can now be ionized. A second position ‘measurement’ of the alpha particle is about to be made.
The quantum measurement laws now tell us that one and only one of the atoms will be ionized. It is selected by pure chance – it can be anywhere in the spot. Once again, the entire wave function that ‘bathes’ the other atoms is instantly destroyed, and a new narrow beam continues outward from the second ionized atom. The same process of ionization, collapse and ‘jet formation’ is repeated at each successive shell. For an alpha particle with sufficient energy, this may happen hundreds or even thousands of times. A track is formed. It has some important features.
First, although it is nearly straight, there are small deflections at nearly all ionizations. It should not be supposed that the deflection occurs where the kink in the track suggests it did. This subtlety is illustrated in Figure 50. At each ionization and collapse a new cone of the wave function is created. It is not unlil the next ionization occurs that any actual deflection angle is selected. Until then, the complete cone of deflection angles is potentially present. As Heisenberg put it in a famous remark, the track is created solely by the fact that we observe the particle.
Second, quantum mechanics makes no predictions about the individual deflection angles. It merely predicts their statistical distribution, according to a law found by Max Born a few months after Schrödinger had created wave mechanics. Its form is determined by the structure of the atoms on which the scattering (deflection) of the alpha particle occurs. It is normally verified by making experiments with many different alpha particles, the statistical distribution being built up by the repetition of many experiments over time. However, in principle it is possible to test the statistical predictions on a single track, especially if it contains thousands of ionizations.
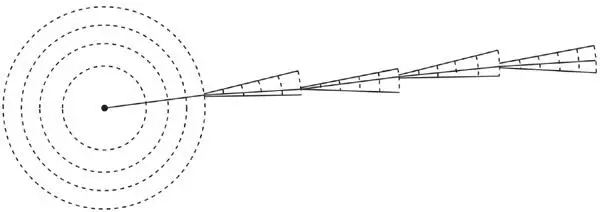
Figure 50.The creation of an alpha-particle track by successive ionizations. After each ionization a wave-function beam spreads out, but it is not until the next ionization occurs that the ‘kink’ is created.
Third, at each ionization the alpha particle loses a fraction of its energy, typically about one part in ten thousand. Since the energy is related to the particle’s wavelength, it becomes progressively longer along the track. Just as diffraction effects are more pronounced for red than for violet light, this means that the deflection angles get progressively larger along the track. The nature of the track changes along its length – it starts to show quite large zigzags.
Bell comments on this first account of track formation that it ‘may seem very crude. Yet in an important sense it is an accurate model of all applications of quantum mechanics.’ Before we consider the second – infinitely more illuminating – account, we need to draw some conclusions and start to develop new ways of thinking about things, above all history.
THE PREREQUISITES OF HISTORY
The central question of this fifth part of the book is this: whence history?
What light does Bell’s first account cast on this question? What are the essential elements that go into the creation of history? Bell’s analysis promises to give us real answers to these questions, since an alpha-particle track can truly be seen as prototypical history. All the elements are there – a unique succession of events, a coherent story and qualitative change as it progresses. It even models birth – when the particle escapes from the radium atom – and demise – when it finally comes to rest. It literally staggers to its death. The laws that govern the unfolding of history are beautifully transparent. They combine, in an intriguing way, causal development – the forward thrust of the track – with unpredictable twists and turns governed only by probability. History is created by what looks like a curious mixture of classical and quantum mechanics – the continuous track and the twists and turns, respectively.
Three distinct factors together create history in this first account. First, the alpha particle emerges from the radium atom in a state that matches geometrical optics. Its wave function propagates outward in perfectly spherical waves of an extremely regular shape and with a very high frequency and short wavelength. This is a perfect example of a semiclassical solution. Hamilton’s ‘light rays’ are the tracks that run radially outward from the radium atom, always perpendicular to the wave-function crests. Each of these tracks is a good simplified model of the one solitary track that eventually emerges.
I mentioned the ongoing saga of geometrical optics. Schrödinger attempted to create history by superimposing many slightly different semiclassical solutions in a wave packet that mimicked particle motion. We can now see that this attempt was doomed to failure, mainly because it attempted to create particle tracks using the quantum-mechanical properties of just one particle in isolation. The interaction of the particle with the environment played no role in Schrödinger’s attempt, but is crucial in the account just given. We cannot begin to think of a track being formed without the atoms waiting to be ionized. Geometrical optics still plays a vital role because the very special semiclassical state ensures that sharply defined beams are created by the process of ionization and collapse.
Читать дальше