As was the case with skin, mucosal surfaces generally have an underlying population of phagocytic cells and immune cells. This mucosal defense system, which is distinct from the system that controls immune cells in blood, lymph nodes, and other organs, is called the mucosa-associated lymphoid tissue (MALT). The mucosal surface of the small intestine is underlain with the gastrointestinal-associated lymphoid tissue (GALT), while the lungs have bronchial-associated lymphoid tissue (BALT) and the upper respiratory tract has nasopharyngeal-associated lymphoid tissue (NALT).
These mucosal defense systems at the interface of the innate and adaptive immune systems are composed of macrophages, T cells, B cells, and M cells (microfold cells that engulf gut lumen contents and present them to underlying antigen-presenting cells). Their primary function is to make secretory IgA (sIgA), an antibody that is secreted into mucus. Antibodies are proteins that bind to specific sites on bacteria or other pathogens. sIgA is thought to increase the stickiness of mucin by attaching to mucin sugars at one end, leaving its two other antigen-binding ends free to bind and trap bacteria trying to reach the mucosal layer. The sIgA-trapped bacteria are then sloughed off along with the mucin. We will return to the role of MALT in chapter 4.
Models for Studying Breaches of Barrier Defenses
Animal models have been widely used to study skin, eye, and mucosal infections. Because some of these animal models involve breaches such as cuts or burns that can be damaging and painful, experimental protocols must include explicit plans to monitor and minimize pain and discomfort to the animals as much as possible and to minimize the number of animals needed to obtain statistically significant results. When available, validated alternative infection models that do not involve animals should be used. In addition, a committee with expertise in animal welfare and experimentation must first approve the rationale for experiments on animals and the detailed protocols themselves before the experiments are performed. Some of the ethical and procedural issues that lead to appropriate animal experimentation are discussed later in chapter 8. For continuity, some of the models used to study eye, skin, and mucosal infections are mentioned here without this context.
One of the earliest models for studying skin infections is the burned-rodent model. A patch of skin on an animal that is anesthetized is shaved and then burned with an alcohol flame. Just as is the case with human burns, bacteria that could not infect intact skin can infect the burned rodent tissue. The eye is another surface of the body that is remarkably resistant to infection. Eye infections of the sort seen in patients who have been careless with contact lenses or have suffered small cuts in the cornea are mimicked by a rabbit model, in which small shallow cuts are made in the cornea of an anesthetized animal’s eye. Both of these models have been used extensively to study infections caused by P. aeruginosa, one of the main causes of burn and eye infections in humans.
In the previous chapter, some unusual lower animal models were mentioned. Caenorhabditis elegans (worms) and Drosophila (flies) are not very useful for studies of skin infections, because the “skin” of these organisms is chitinous rather than epithelial. The zebra fish is a better model, especially for studies of the mucosal defenses. More recently, infection models have been developed based on tissues from, for example, chicken embryos. Rodents have been widely used to investigate pathogens, such as Salmonella, that bind to the intestinal mucosa. In rodents, these pathogens can sometimes cause more invasive infections than they cause in humans, but the interaction between the bacteria and the mucosa can nonetheless be followed even in these cases. A rodent model has been developed in which autoclaved feces, inoculated only with the bacterium of interest, are implanted in the intra-abdominal area of the rodent to mimic the effects of surgical penetration of the colonic mucosa.
The impact of toxins, such as diarrhea-causing toxins, on the small intestine can be monitored by the rabbit ileal loop model ( Figure 2-10). In this model, the small intestine of an anesthetized rabbit is tied off into 5- to 10-cm sections by suture, the toxin or toxin-producing bacterium is injected into one of the sections (loops), and the organ is placed back into the peritoneal cavity. Many diarrheal toxins cause water to be lost by the intestinal tissues into the lumen of the gut, and this can be observed by a swelling of the section into which the toxin was injected. After 12 to 24 hours, the animal is sacrificed and the loop length and fluid volume (in ml/cm) are measured as readout. Distension (i.e., swelling) of the ileal loop section indicates release of the fluid into the lumen of the segment as a result of toxin action.
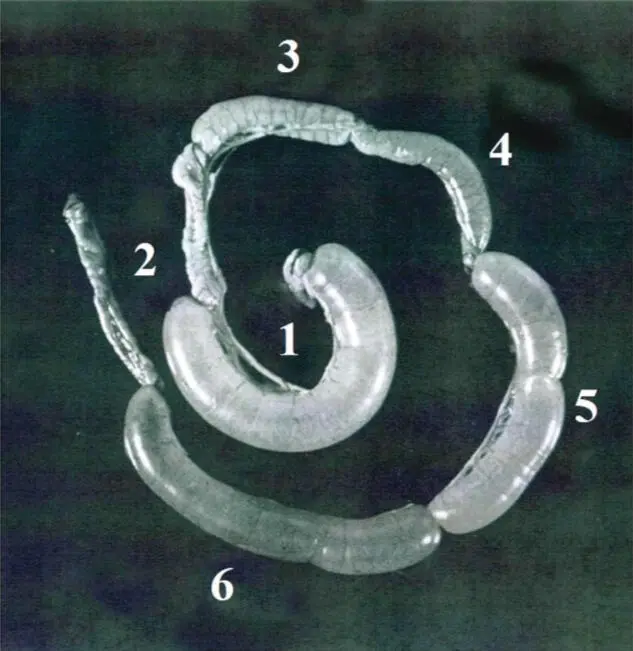
Figure 2-10. The rabbit ileal loop model of diarrheal disease. Shown are tied-off segments (loops) of rabbit ileal injected with culture filtrates from an E. coli strain producing cholera-like toxin that induces diarrhea. Loop 1 was injected with positive control solution of cholera toxin, loop 2 with negative control solution of phosphate-buffered saline, and loops 3 through 6 with increasing amounts of E. coli culture filtrates. After overnight exposure in the animal, the animal is sacrificed and the ileal loops are removed and examined for distension (swelling). Reproduced from Sack RB. 2011. Indian J Med Res 133:171–180, with permission.
Genetically engineered mice called transgenic mice or knockout mice are being increasingly used in experiments to probe the interaction of the normal microbiota and the intestinal mucosal cells because they have genes that have been altered or disrupted. Unexpectedly, some of the mice designed originally for studies of the immune system that were missing genes encoding the cytokines, interleukins IL-1 and IL-10 (see chapter 3), proved to be good models for a type of intestinal inflammation called inflammatory bowel disease (IBD). The presence of the normal bacterial microbiota of the colon seems to be responsible for the inflammatory bowel condition seen in some of these mice.
These examples are given to provide an introduction to the types of animal models that are available for studying the protective features of skin and mucosa and the consequences of breaching these barriers. Additional animal models used in connection with studying bacterial diseases, as well as alternatives to animal models such as mammalian host cells cultured in vitro in the laboratory, will be described in chapter 8and other chapters covering specific types of infectious diseases.
SELECTED READINGS
Bik EM, Eckburg PB, Gill SR, Nelson KE, Purdom EA, Francois F, Perez-Perez G, Blaser MJ, Relman DA. 2006. Molecular analysis of the bacterial microbiota in the human stomach. Proc Natl Acad Sci USA 103:732–737. [PubMed] [CrossRef]
Cooper GM, Hausman RE. 2007. The Cell—A Molecular Approach, 4th ed. ASM Press, Washington, DC.
Hooper LV. 2004. Bacterial contributions to mammalian gut development. Trends Microbiol 12:129–134. [PubMed] [CrossRef]
Hooper LV, Wong MH, Thelin A, Hansson L, Falk PG, Gordon JI. 2001. Molecular analysis of commensal host-microbial relationships in the intestine. Science 291:881–884. [PubMed] [CrossRef]
Mirmonsef P, Spear GT. 2014. The barrier to HIV transmission provided by genital tract Lactobacillus colonization. Am J Reprod Immunol 71:531–536. [PubMed] [CrossRef]
Mukherjee S, Hooper LV. 2015. Antimicrobial defense of the intestine. Immunity 42:28–39. [PubMed] [CrossRef]
Читать дальше