Genome Editing in Drug Discovery
Здесь есть возможность читать онлайн «Genome Editing in Drug Discovery» — ознакомительный отрывок электронной книги совершенно бесплатно, а после прочтения отрывка купить полную версию. В некоторых случаях можно слушать аудио, скачать через торрент в формате fb2 и присутствует краткое содержание. Жанр: unrecognised, на английском языке. Описание произведения, (предисловие) а так же отзывы посетителей доступны на портале библиотеки ЛибКат.
- Название:Genome Editing in Drug Discovery
- Автор:
- Жанр:
- Год:неизвестен
- ISBN:нет данных
- Рейтинг книги:5 / 5. Голосов: 1
-
Избранное:Добавить в избранное
- Отзывы:
-
Ваша оценка:
- 100
- 1
- 2
- 3
- 4
- 5
Genome Editing in Drug Discovery: краткое содержание, описание и аннотация
Предлагаем к чтению аннотацию, описание, краткое содержание или предисловие (зависит от того, что написал сам автор книги «Genome Editing in Drug Discovery»). Если вы не нашли необходимую информацию о книге — напишите в комментариях, мы постараемся отыскать её.
A practical guide for researchers and professionals applying genome editing techniques to drug discovery Genome Editing in Drug Discovery,
Genome Editing in Drug Discovery
Genome Editing in Drug Discovery
Genome Editing in Drug Discovery — читать онлайн ознакомительный отрывок
Ниже представлен текст книги, разбитый по страницам. Система сохранения места последней прочитанной страницы, позволяет с удобством читать онлайн бесплатно книгу «Genome Editing in Drug Discovery», без необходимости каждый раз заново искать на чём Вы остановились. Поставьте закладку, и сможете в любой момент перейти на страницу, на которой закончили чтение.
Интервал:
Закладка:
Saša Šviković
Genome Engineering, Discovery Sciences, BioPharmaceuticals R&D, AstraZeneca, Gothenburg, Sweden
3.1 Introduction
Over the course of billions of years of evolution, prokaryotes have developed multiple ways to stay ahead of their fiercest foes, mobile genetic elements. These defense systems include a variety of nucleases, DNA‐modifying enzymes, chemical inhibitors, cell death‐inducing signaling pathways, and some not yet fully understood protection systems (Bernheim and Sorek 2020; Hampton et al. 2020). These systems are incredibly diverse and widespread among prokaryotes, and studies into how these systems function ultimately led to the rise of new tools that have revolutionized biomedical sciences. Similarly to how the discovery of an innate bacterial immune system mediated by restriction endonucleases has ushered a revolution in biotechnology (Roberts 2005), the relatively recent discovery of CRISPR‐Cas systems, an adaptive immune component of prokaryotic defense systems, and their application in genome engineering has completely changed the way how research in life sciences is done. In this Chapter, we will address the basics of CRISPR biology, the diversity and current classification schemes, and how the mechanistic understanding of these diverse systems can give rise to novel tools for biomedical science.
3.2 CRISPR Biology in a Nutshell
CRISPR, or Clustered Regularly Interspaced Short Palindromic Repeats, represents an adaptive immune system of microbes, able to adapt in response to invasions by mobile genetic elements such as bacteriophages, plasmids, and transposons. The locus encoding for the components of the CRISPR system was discovered as an array of palindromic repeats interrupted by a 20–40 nt sequence downstream of the iap gene in Escherichia coli (Ishino et al. 1987; Nakata et al. 1989). Thanks to the increasing availability of sequences from the microbial species, the structure of CRISPR loci, with many properties relating to their function, was uncovered (Mojica et al. 2000; Jansen et al. 2002).
The identifying trait of the CRISPR locus in an array of alternating identical repeats, interleaved by unique spacer sequence( Figure 3.1a), with extreme interspecies diversity in sequence and size, usually in the range of 20–72 bp (Grissa et al. 2007). Bioinformatic analysis of spacer sequences within CRISPR arrayshas shown a striking homology to various sequences found in bacteriophages and plasmids (Bolotin et al. 2005; Mojica et al. 2005; Pourcel et al. 2005), which was an early clue that CRISPR systems act as a defense mechanism against mobile genetic elements (Makarova et al. 2006). The collection of the spacers within a microbial species is extremely diverse and dynamic (Weinberger et al. 2012), as the microbes can acquire new spacers upon neutralizing the infection (Barrangou et al. 2007; Marraffini and Sontheimer 2008). The spacer acquisition represents the adaptive component of this immune system, as this confers resistance to reinfection by the same mobile genetic element.
In most species, repeat monomers vary between 23 and 47 bp in length (Godde and Bickerton 2006), and in most species consist of partially palindromic sequences, able to form stable secondary structures (Kunin et al. 2007). Related species can have similar repeat sequences, but the overall bacterial and archaeal sequence diversity of both spacers and repeats is great.
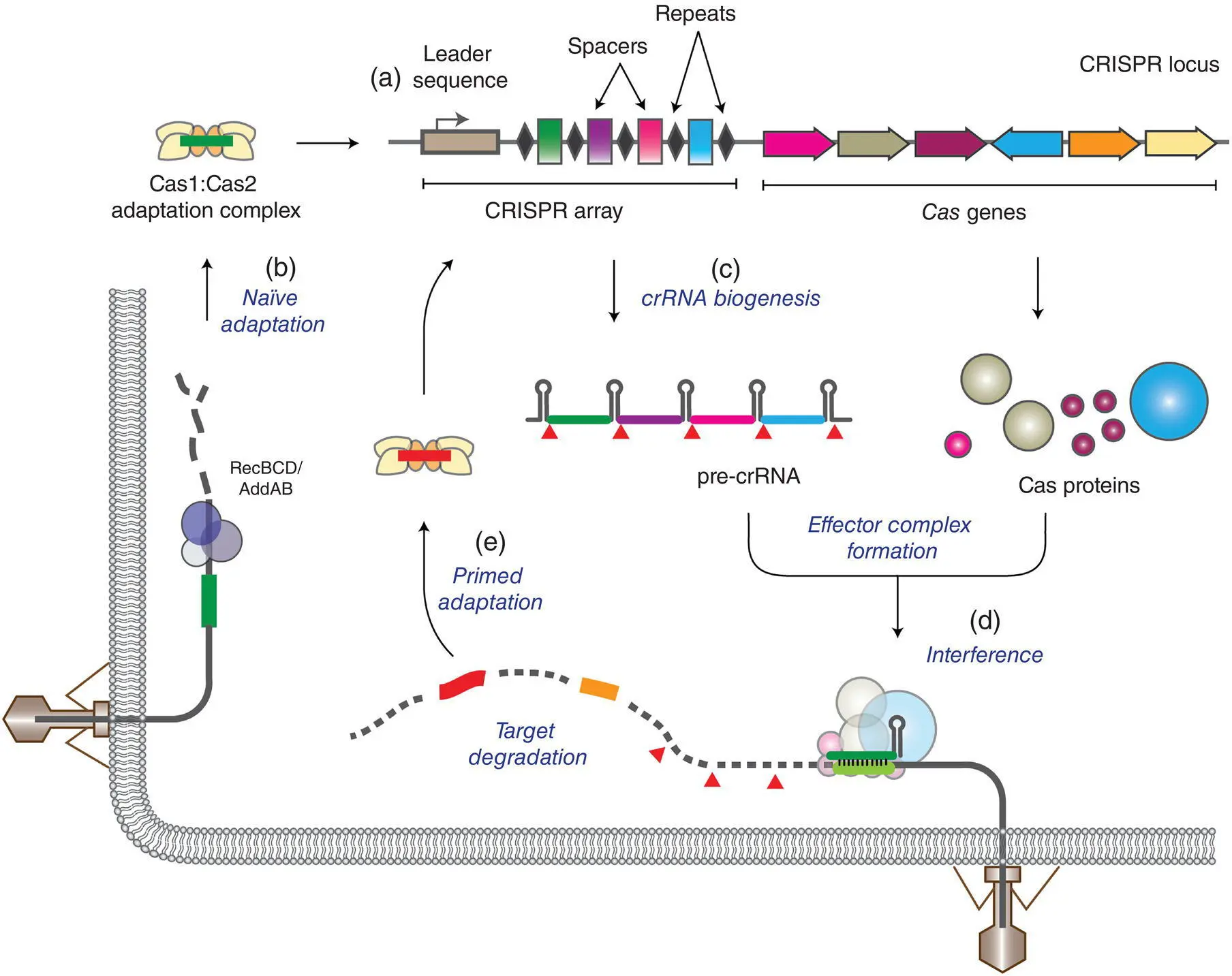
Figure 3.1 Phases of CRISPR‐mediated immune response. ( a) The simplified architecture of an idealized CRISPR locus, containing a CRISPR array consisting of a promoter‐containing leader sequence and spacer and repeat sequences (depicted on the left) and several different cas genes (depicted in different colors on the right), which generate functional components of the CRISPR‐Cas system. During the infection by a mobile genetic element (depicted as a bacteriophage here), invading DNA can be processed by the RecBCD/AddAB systems so that it can be integrated as a spacer by the Cas1:Cas2 adaptation complex as a part of the naïve adaptation phase ( b). In the expression phase ( c), the CRISPR array is transcribed into pre‐crRNA, which is further processed to crRNA and paired with Cas proteins to form a functional effector complex. Upon a reinfection with phage carrying the same or similar protospacer sequence, the effector complex recognizes the sequence ( d) and induces the degradation of the target. Suitable fragments generated during the interference phase can be used as spacers in the primed adaptation phase ( e), updating the CRISPR array with the most recent version of suitable spacers.
Immediately adjacent to the CRISPR array is an AT‐rich element, most frequently between 100 and 400 bp long (Jansen et al. 2002; Alkhnbashi et al. 2016). It was subsequently shown that this sequence, termed leader sequence, contains the promoter used to direct transcription of the CRISPR array ( Figure 3.1a) and determines the transcriptional start site (Pougach et al. 2010; Pul et al. 2010). Transcription of the CRISPR locus and its subsequent processing ultimately gives rise to CRISPR RNA ( crRNA), which provides specificity to the CRISPR systems and guides the degradation of the invading nucleic acids (Brouns et al. 2008). Much like the repeats, leaders are up to 80% identical within a genome, but quite dissimilar among species.
CRISPR arrays are often surrounded by a collection of conserved protein coding genes, termed CRISPR‐associated or cas genes; these genes encode for the protein components of the CRISPR immunological systems. The groups of protein coding genes vary dramatically in composition, order, and sequence between species, underpinning the diversity of CRISPR systems, and their modes of action. Indeed, the complement of cas genes, together with the structure of the CRISPR locus is the basis for CRISPR classification (Makarova et al. 2019). Furthermore, many genes not evolutionary related to cas genes can also be found within the CRISPR loci. These genes often encode for proteins providing ancillary functions, or are evolutionary relicts and do not have a role attributed to them yet, and some loci also encode for noncoding RNA. Furthermore, there might be multiple CRISPR loci within a single genome: E. coli has four loci (Touchon and Rocha 2010) and Methanocaldococcus jannaschii has up to 18 (Lillestol et al. 2006), but rarely more than one or two are active simultaneously (Horvath et al. 2008).
All the components of CRISPR loci function together to provide an adaptive immune response. The CRISPR immune response can be divided into three phases ( Figure 3.1):
1 Adaptation (spacer acquisition, or immunization). On a rare occasion during infection by a bacteriophage or other mobile elements, suitable pieces of invaders genome can be snatched up by the microbe’s defense machinery and integrated into CRISPR array as a spacer by the adaptation machinery ( Figure 3.1b). This acquisition of not previously encountered spacers is known as naïve adaptation. Once acquired, the spacer acts as a heritable record of immunization and will restrict mobile elements which have the exact or similar sequence through the next stages of the immune response.
2 Expression (crRNA biogenesis). Once acquired, the spacer can be utilized to fight future infections ( Figure 3.1c). The whole CRISPR array is transcribed from the promoter located within the leader sequence, producing a pre‐crRNA transcript. With the help of Cas proteins, other small RNA molecules, or the host’s machinery, pre‐crRNA is processed into mature crRNA, which are paired with the effector Cas proteins to form a functional effector complex that confers immunity.
Читать дальшеИнтервал:
Закладка:
Похожие книги на «Genome Editing in Drug Discovery»
Представляем Вашему вниманию похожие книги на «Genome Editing in Drug Discovery» списком для выбора. Мы отобрали схожую по названию и смыслу литературу в надежде предоставить читателям больше вариантов отыскать новые, интересные, ещё непрочитанные произведения.
Обсуждение, отзывы о книге «Genome Editing in Drug Discovery» и просто собственные мнения читателей. Оставьте ваши комментарии, напишите, что Вы думаете о произведении, его смысле или главных героях. Укажите что конкретно понравилось, а что нет, и почему Вы так считаете.